« Back to In-Space Production Applications
Advanced Manufacturing and Materials
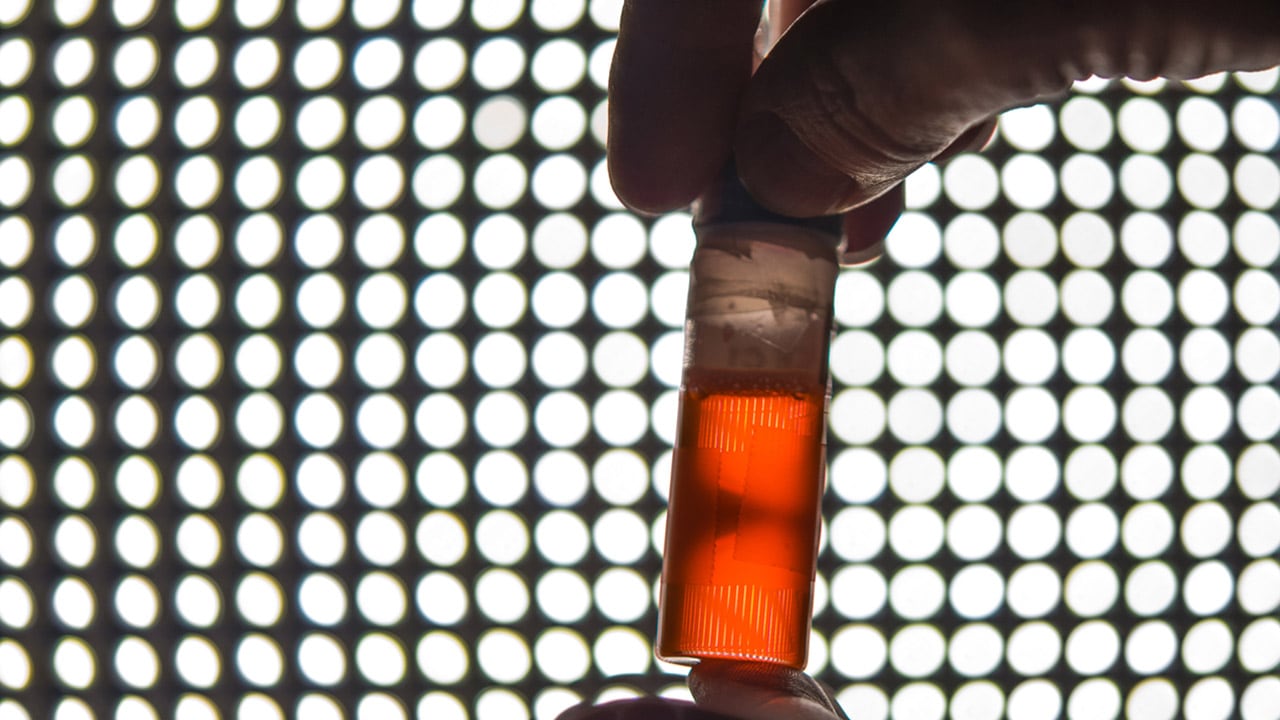
In-Space Production Applications: Advanced Manufacturing and Materials
Projects within this area develop next-generation production methods, improve understanding of mechanisms involved in material transformations, advance fundamental materials discovery, and test processes or manufacturing methods of novel design and synthesis pathways. Previous researchers have studied combustion, complex fluid flow, interfacial phenomena, materials degradation, and in-orbit manufacturing, among others.
Advanced materials and manufacturing:
- Metal-organic frameworks
- Exotic glasses and fibers
- Ceramics
- Containerless processing
- Low-mass materials
- Multiphase materials
- Superalloy casting
- Semiconductors
Earth’s gravity confounds precise measurements of the thermophysical properties of materials and their interactions through the effects of convection, buoyancy, sedimentation, and contact with the container in which their properties are measured.
MicrogravityThe condition of perceived weightlessness created when an object is in free fall, for example when an object is in orbital motion. Microgravity alters many observable phenomena within the physical and life sciences, allowing scientists to study things in ways not possible on Earth. The International Space Station provides access to a persistent microgravity environment. enables research to observe, model, and exploit underlying physical dynamics that are typically masked by gravity-dependent phenomena in terrestrial studies of combustion, fluids, complex fluids (such as colloids and non-Newtonian fluids), soft matter, and hard materials. For example, breakthroughs in studies related to fluid dynamics, phase separation, and the fundamental internal structure of fluids may accelerate formulation chemistry; nanofluidics technologies; water and energy conservation solutions; enhanced energy generation, transfer, and storage; and development of novel materials and better manufacturing processes on Earth.
Materials research in microgravity can also reveal fundamental mechanisms in crystallization, solidification, network formation, and phase transitions that are obscured in Earth’s gravity. A deeper understanding of these mechanisms, for example, contributed to the terrestrial development of improved superalloys, stabilized colloid-based products, and better casting processes. Additionally, fundamental and applied microgravity studies may have human health applications; for example, studies on interfacial phenomena promise to advance strategies for prevention of the microbial contamination of food and microbiologically influenced corrosion (or microbially induced corrosion) of materials.
In addition to microgravity, the extreme conditions of the space environment are demonstrably hostile to both materials and the components that they comprise. While Earth-based experiments are often conducted under artificial conditions to simulate extreme operational conditions, space is the ultimate test objective with simultaneous exposure to multiple environmental extremes, providing a mechanism for rapid failure mode and effect analysis.
Why conduct this research in space?
In microgravity, the absence of gravity-driven phenomena enables the production of a wide range of new materials.
For example:
- Removing sedimentation and buoyancy enables the production of unique alloys and compositions.
- Surface tension processes may eliminate voids and ensure continuous contact between dissimilar materials.
- Lack of convection provides quiescent environments that may remove or minimize defects.
Examples of In-Space Production Applications Advanced Manufacturing and Materials Projects
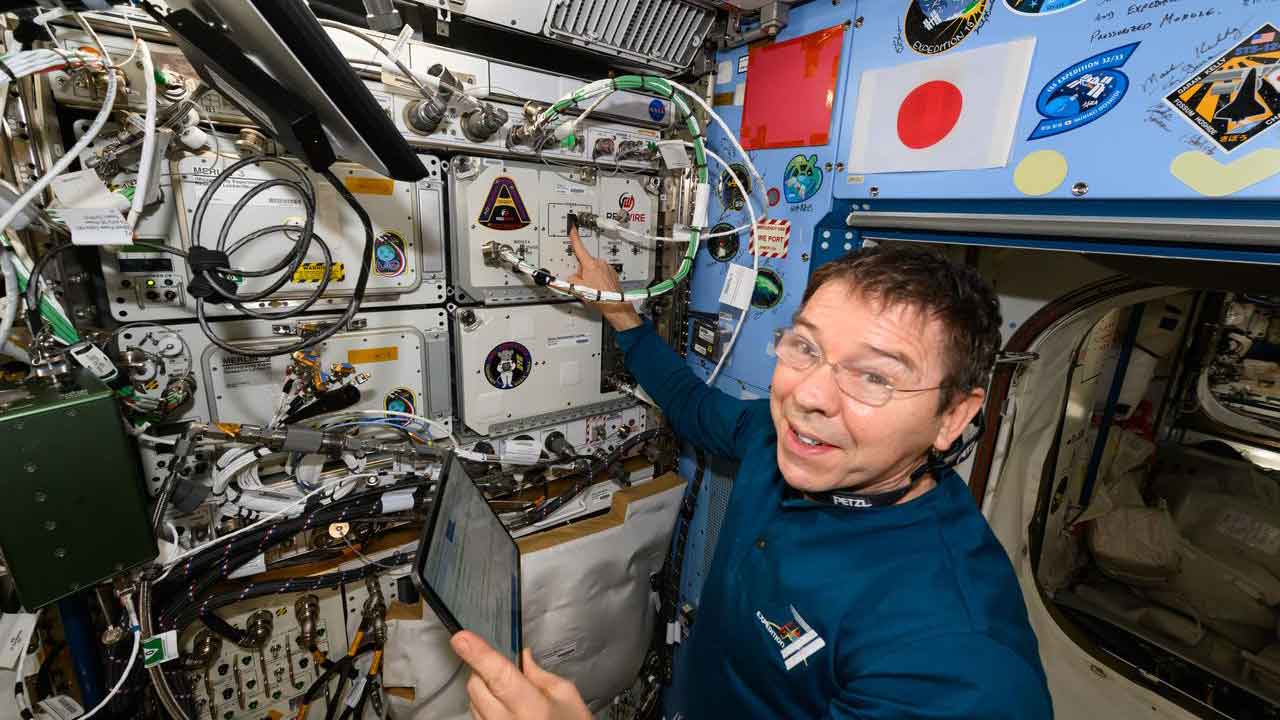
Media Credit: NASANational Aeronautics and Space Administration
Metal-Organic Frameworks
Metal-organic frameworks (MOFs) are a novel class of porous materials consisting of metal ions or clusters in coordination within a framework of repeating organic structures. They are a product of a mixed process that includes chemical transformation and crystallization. These materials can be difficult to produce but are potentially useful for a variety of applications, including use as a catalyst in the acceleration of chemical transformations or for components in optical systems, and most notably, systems designed to adsorb gases with high specificity and efficiency.
Mixed crystal systems like MOFs that involve both a chemical transformation and crystallization have the potential to impact various industries, including the chemical, energy, and materials production industries. Space is beneficial for this research because microgravity improves crystallization outcomes and because the reduction of gravity-driven sedimentation and buoyancy helps prevent stratification.
Research projects in this area could also fall under the category of inorganic crystal growth, which represents an opportunity to create products of great value using a rich history of experience in growing crystals in space.
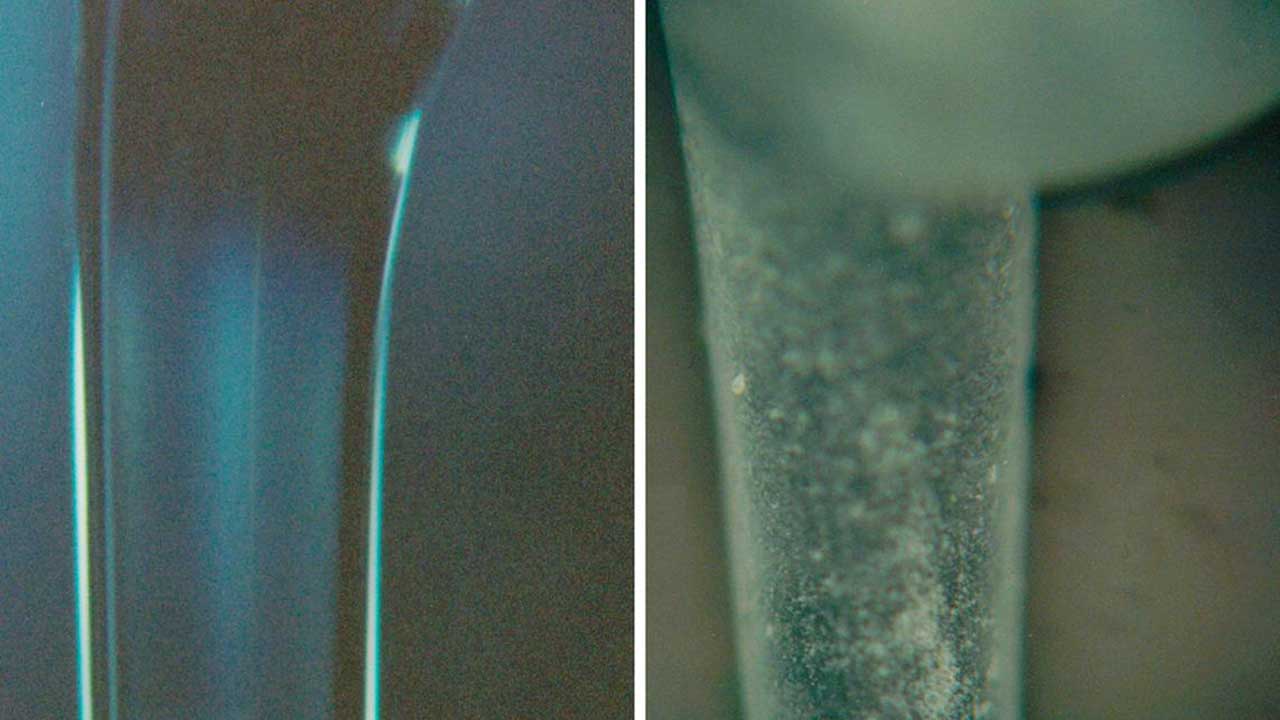
Media Credit: NASA
Exotic Glasses and Fibers
Exotic glasses and fibers enable wavelength-specific laser and sensor applications for defense, medicine, and communications applications. Research in this area informs novel formulations of difficult-to-manufacture glasses for advanced applications on Earth. Metrics for gauging overall quality of the fiber produced include fiber length, attenuation, % rare earth doped, and strength.
For example, fluoride optical fibers, such as ZBLAN, have demonstrated a 10- to 100-fold signal loss reduction compared with traditional silica optical fibers. High-quality fluoride optical fibers could dramatically improve the cost and efficiency of communications systems and the internet. However, high-quality fluoride optical fibers are difficult to produce on Earth because imperfections that occur during manufacturing on Earth prevent the fibers from achieving this reduction in signal loss.
Microgravity suppresses crystallization in ways that may allow significantly fewer defects in exotic glasses and optical fibers that are difficult to produce on Earth. Microgravity also eliminates sedimentation and buoyancy, which should result in better distribution of constituent materials.
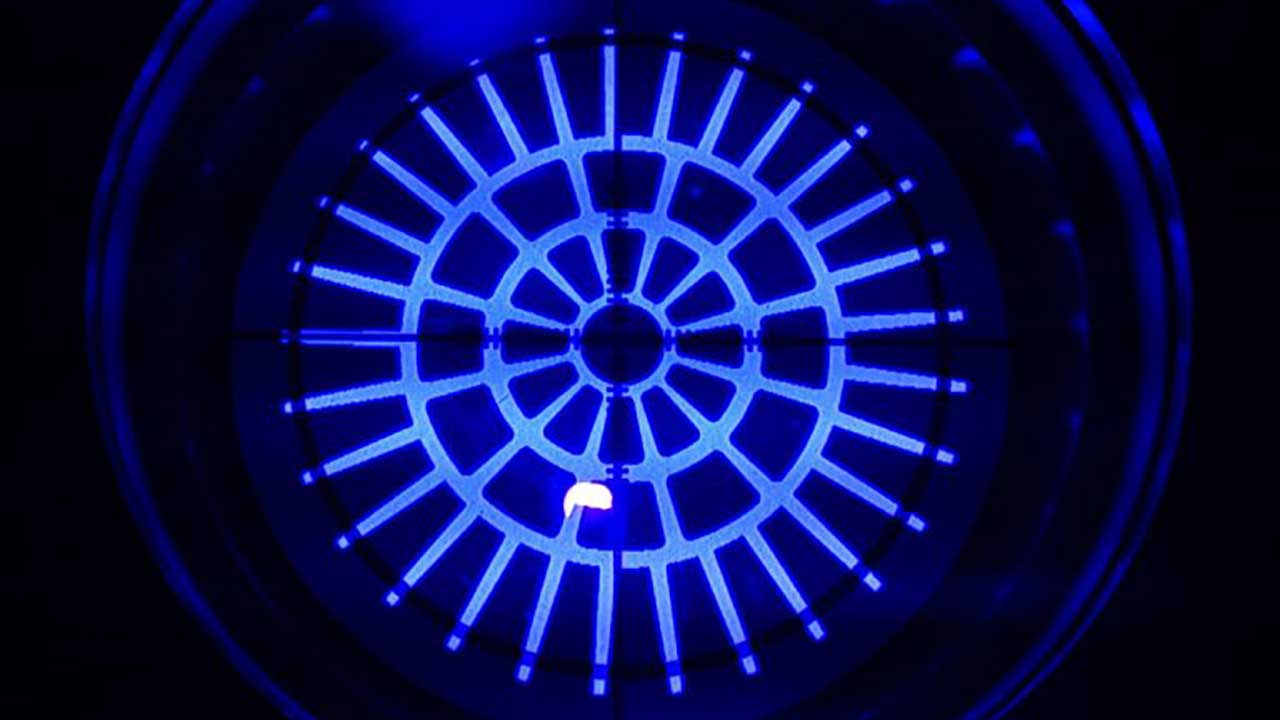
Media Credit: Redwire Space
Ceramics
New enabling technologies on the ISSInternational Space Station like Redwire’s Ceramic Manufacturing Machine can enable the application of new techniques such as stereolithography (a form of additive manufacturing where layers of material are added using a photosensitive mixture activated by lasers) of pre-ceramic resins. Because three-dimensional structures do not slump or flatten out in microgravity as they would when exposed to terrestrial gravity forces, space-based ceramic production can improve the outcomes when structural support is not possible. Ceramic production in microgravity may also improve fine detail of delicate ceramic structures, leading to products with applications in areas where strength and fine design detail are required. Examples include turbines, pump and valve systems, and machine parts requiring chemical and heat resistance.
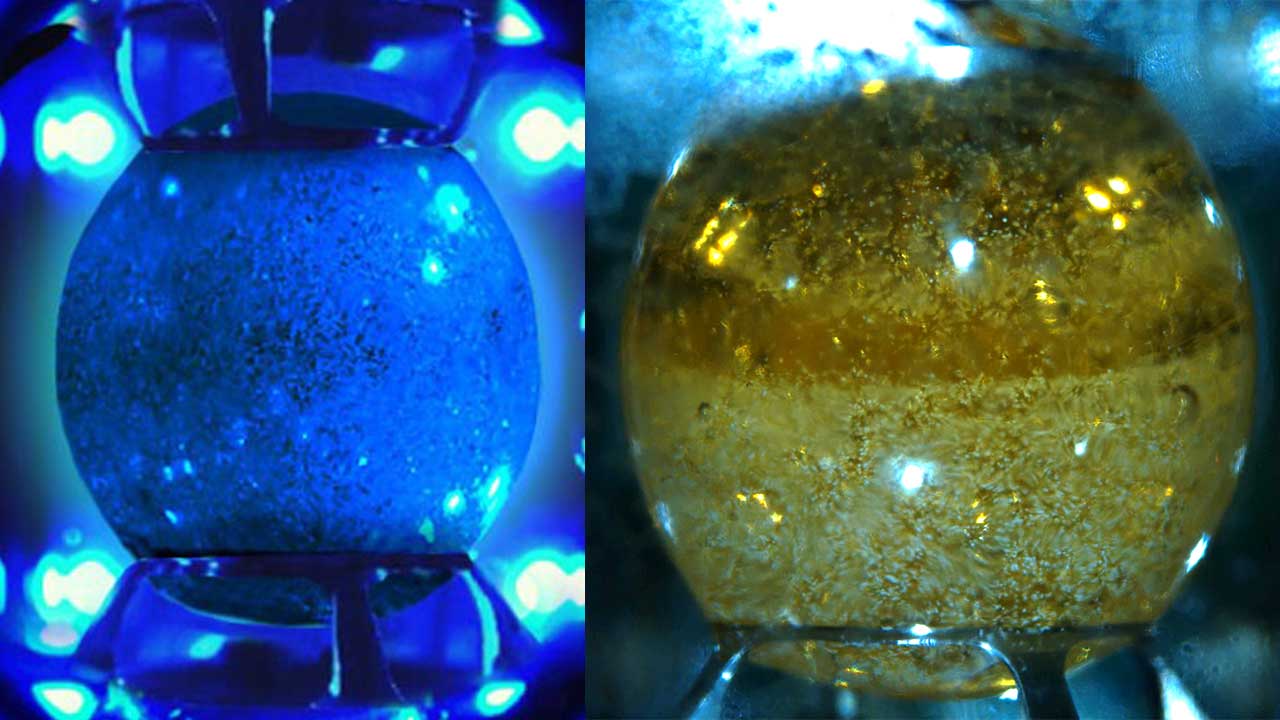
Media Credit: Joe Adam
Containerless Processing
Containerless processing (processing of materials where the substances are not touching the top bottom or sides of the container in which the process occurs) can eliminate contamination, erroneous reactions, heterogeneous nucleation, and surface tension-driven segregation. Researchers have taken advantage of this effect in different ways. For example, metals tend to nucleate and form new crystalline structures at the site of contact with the surface of the crucible. A smelter that can heat the metal but not have physical contact with the sample would lead to new structures. There are built-for-purpose systems (systems designed to perform containerless processing) on the ISS, including the two levitator furnaces.
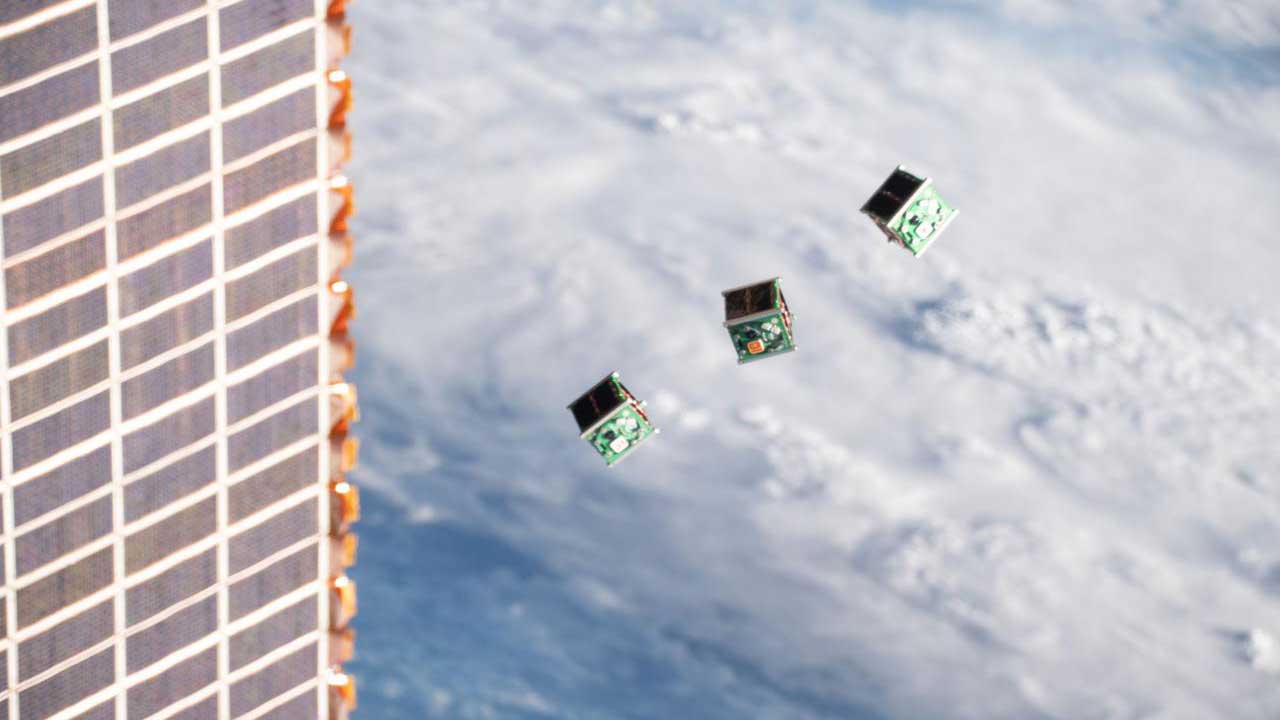
Media Credit: NASA
Metamaterials and Low-Mass Materials
This is a broad class of materials engineered to have unique properties, not found in naturally occurring materials, related to their structure and chemical makeup. They are often very light (low mass or density) and can have very intricate structures that make them good insulators and allow them to interact with and focus light, heat, sound, magnetic and electrical waves in unique ways. This class of materials also has applications in the production of very light satellite structures and components that can be produced in orbit and do not need to be built sturdy enough to withstand launch stress or gravity.
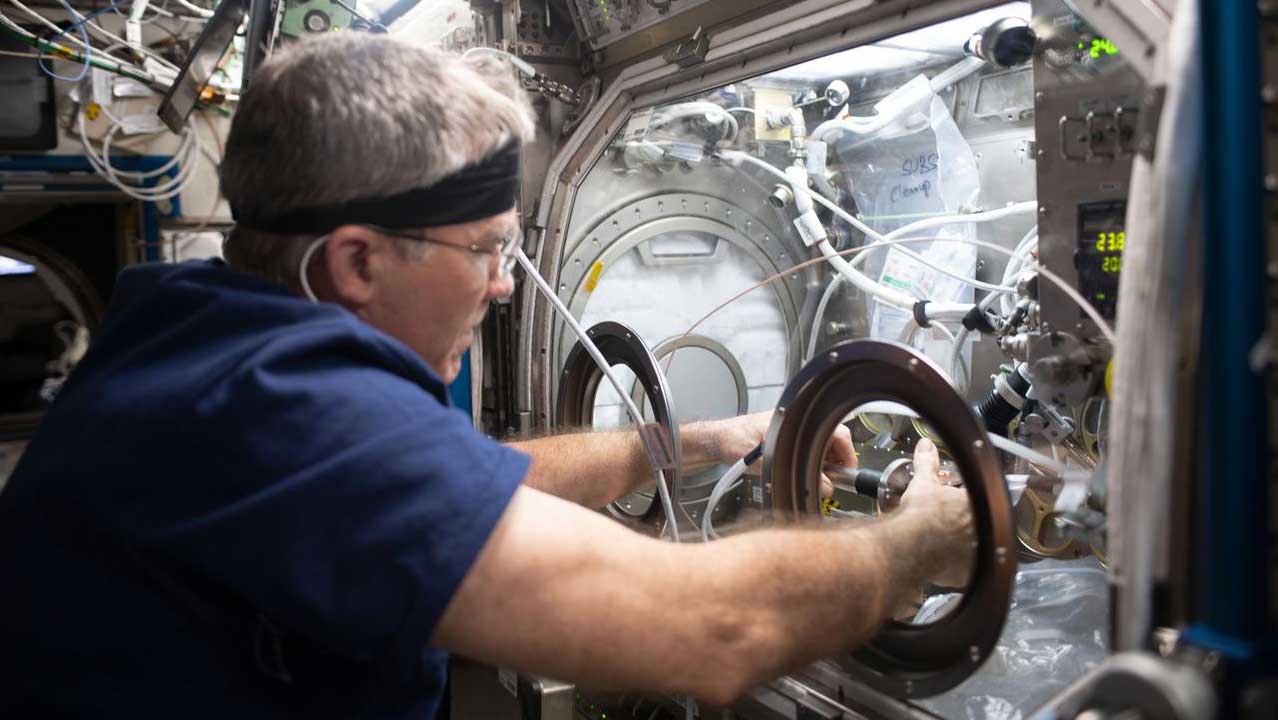
Media Credit: NASA
Multiphase Materials
Multiphase materials exist as a combination of gaseous, liquid, or solid phases that self-assemble into structures. However, gravity can disrupt the self-assembly through sedimentation, stratification, and buoyancy-driven phase separation. This causes defects and anisotropy (properties change with respect to direction), which can be detrimental to material properties. Production of multiphase materials in microgravity may lead to improved uniformity of meso-scale structuring. This can improve mechanical, thermal, and transport properties, in addition to increasing surface area. Potential multiphase materials may include aerogels, bijels, hydrogels, liquid crystals, colloidal crystals, and block copolymer self-assembly.
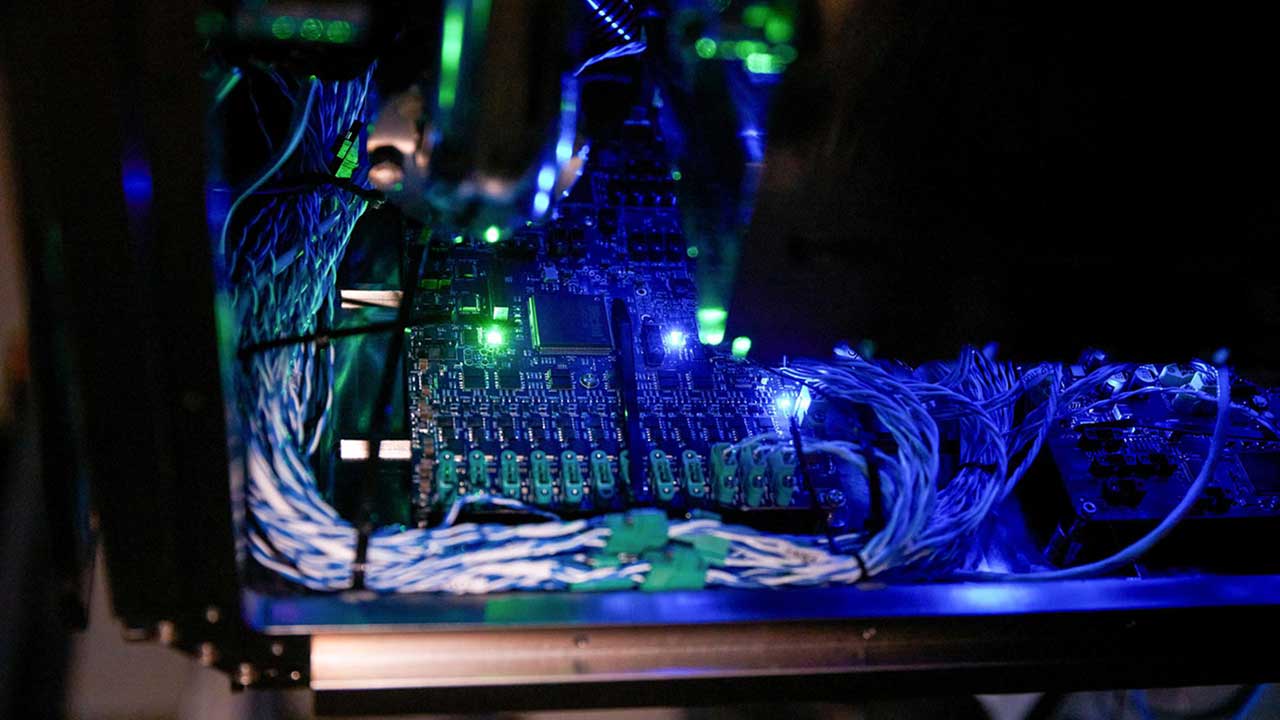
Media Credit: Redwire Space
Super-Alloy Casting
Super alloys are metal alloy systems made up of elemental mixtures that would be difficult, if not impossible, to prepare in a gravity environment and can possess unique properties. Next-generation metal processing and casting systems on the ISS will be able to achieve higher temperatures than the current SUBSA furnace on station. These new systems will enable the manipulation of alloys that require higher temperatures than previously available for processing in orbit, further opening the field of space-based metallurgy and the production of new metal alloys.
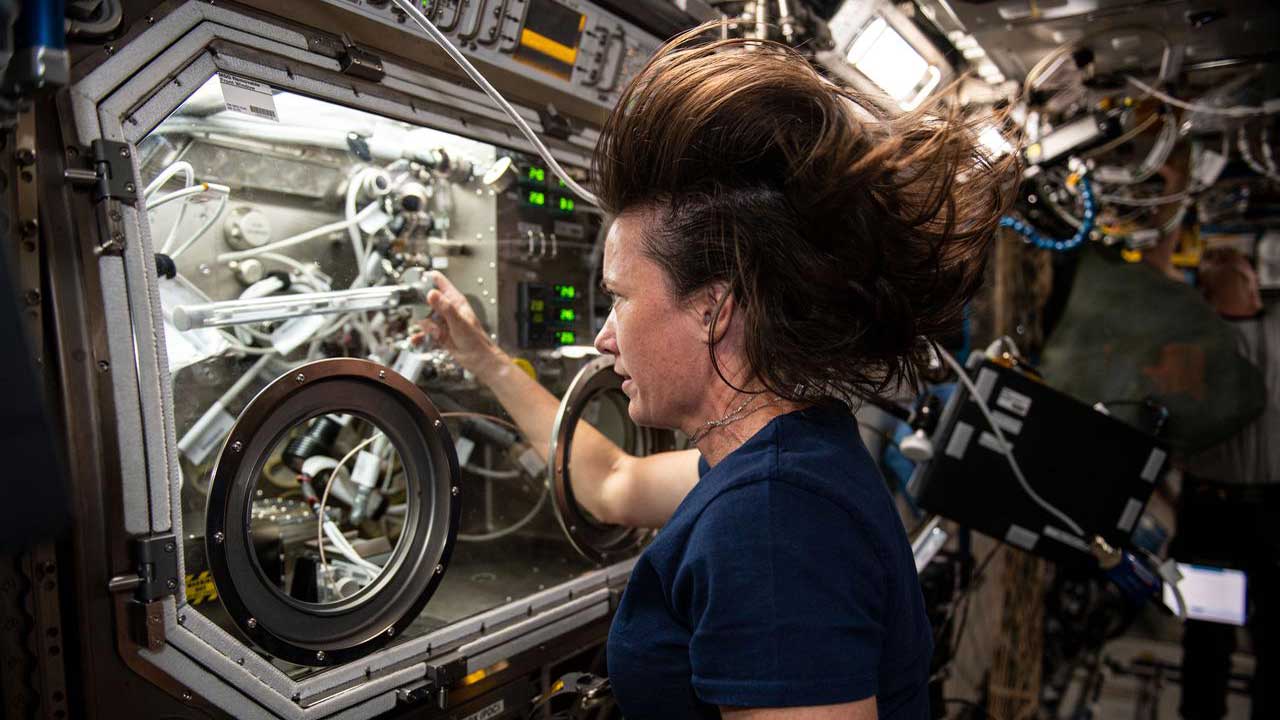
Media Credit: NASA
Semiconductors
Microgravity can enable the production of thin-layer systems with high fidelity that will facilitate the manufacture of next-generation microprocessors. Thin-layer deposition using graphene, for example, could not only lead to improved semiconductor performance but will help us understand the effects of gravity-induced convection on the quality of nanomaterial production and will serve as a model for long-term improvements in nanomaterial manufacturing.