Finding a Key to the Heart: Stem Cells in Microgravity Could Improve Cardiac Repair
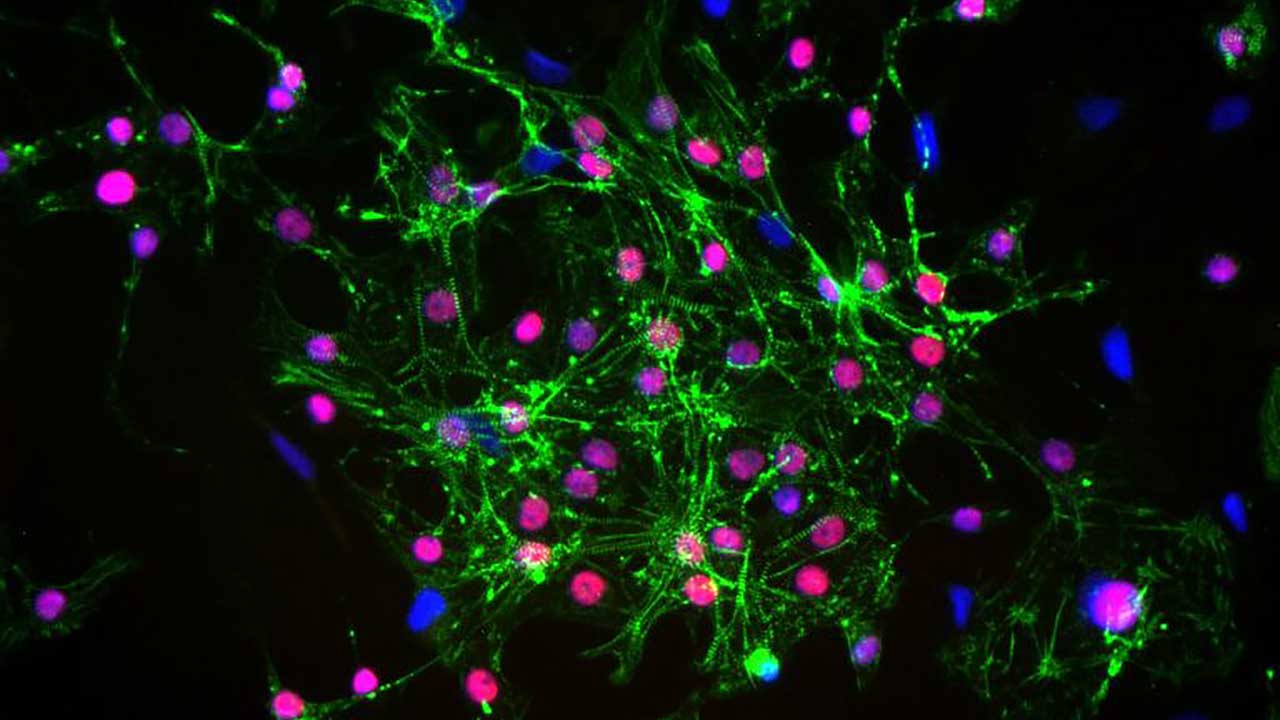
Detection of cardiac structural proteins (stained green) and cardiac transcription factor (stained red) in cells following cryopreservation and carbon dioxide-independent culture. The nuclei are stained blue.
Media Credit: Antonio Rampoldi, Cardiomyocyte Stem Cell Laboratory, Emory University School of Medicine
August 13, 2021 • By Eric Rogers, Staff Writer
MicrogravityThe condition of perceived weightlessness created when an object is in free fall, for example when an object is in orbital motion. Microgravity alters many observable phenomena within the physical and life sciences, allowing scientists to study things in ways not possible on Earth. The International Space Station provides access to a persistent microgravity environment. could be the key that unlocks the full potential of cardiac stem cell growth, according to a team of researchers from Emory University. The team leveraged the International Space Station (ISSInternational Space Station) U.S. National Laboratory to study microgravity’s effects on stem cells and their differentiation into cardiomyocytes (heart muscle cells).
The trouble is, culturing stem cells in low Earth orbit(Abbreviation: LEO) The orbit around the Earth that extends up to an altitude of 2,000 km (1,200 miles) from Earth’s surface. The International Space Station’s orbit is in LEO, at an altitude of approximately 250 miles. is a challenge. Even getting the cells there alive and back in one piece is difficult. Luckily, new developments from an Emory team led by Chunhui Xu, an associate professor in the Emory University Department of Pediatrics, have made that process easier—and produced results that eventually could fast-track the application of stem cells from the research lab to the clinic.
Using a meticulously designed cryopreservation process and a novel cell culture technology developed specially for the mission, Xu and her team sent a series of cell cultures containing groups of cardiac progenitor cells—advanced stem cells destined to become fully functioning heart cells—to the ISS. The samples were cultivated on station for 22 days before returning to Earth.
The results: the progenitor cells not only grew and differentiated into beating heart cells in microgravity—they grew faster and survived better than their counterparts cultured onboard the ISS in a centrifuge configured to simulate normal Earth gravity (1g).
“We were very excited to see a significant difference between the 1g control and the microgravity cultures,” Xu said.
Some of the team’s findings were published earlier this year in the journal Biomaterials and were the subject of a recent virtual event that included a discussion with astronaut Jessica Weir, who worked on the investigation onboard the ISS.
Exciting Results Pave the Way to Space
While Xu was aiming for space from the start, the first signs her team was on to something came from the lab.
In a preliminary ground validation study in 2013, the Emory team cultivated 3D liquid-batch stem cell cultures for three days in simulated microgravity on the ground, using specialized lab equipment. The process produced mature cardiomyocytes with 99% purity, at a rate eight times higher than standard 2D cell culture techniques.
It was evidence that microgravity conditions, in combination with other techniques, could enhance the growth and purity of heart cells developed from cardiac progenitors. The result was exciting, Xu said, as it hints at a potential breakthrough for future clinical applications of stem cells.
“The heart cells generated from these stem cells have numerous applications, one of which is trying to repair a damaged heart. However, to do this, we need about a billion cells for each patient, and there are so many patients out there,” she said. “So one of the challenges in the field is, how can we generate high-quality cells in a very efficient way?”
The positive results were likely brought on by changes in gene expression, biochemistry, and cellular interaction, induced by the functional lack of gravity in the simulator, Xu said. Attempting to replicate—and possibly enhance—the results in the true microgravity of space was the natural next step.
“In space, there is no shear stress to the cells. They’re kind of free-floating. That’s something we cannot truly duplicate on Earth,” Xu said.
The Challenge of Growing Heart Cells in Space
As Xu and her team learned, doing an experiment in space was not as simple as loading stem cells on a rocket and sending them into low Earth orbit.
For one thing, there was the issue of timing. Xu’s experiment required the cells to be in a certain stage of development, a brief intermediate phase between heart cell and undifferentiated stem cell. A delayed launch or other demands on crew availability could result in missing that critical window.
To address this, Xu and her team experimented with cryopreservation techniques that could temporarily halt cellular development and maximize recovery of live cells on arrival to the ISS. They found success through a process that involved precooling the cells at 4˚ C for a short time before storage at -80˚ C. A chemical additive that acts as a cryopreservant, augmented with a survival-enhancing protein, also improved recovery.
The novel method also had other benefits. Hitting pause on development meant the team could freeze the cultures well in advance, granting time to conduct quality control and ensure that only the healthiest cells made it to space. Additionally, the cryopreservant could act as a buffer against physical shock, further enhancing survival by cushioning cells from the harsh g-forces of launch.
Another challenge of conducting the experiment in space was the growth medium. Typical cell cultures require the addition and careful monitoring of carbon dioxide in the liquid to modulate the pH balance of the substrate. However, the incubation facility onboard the ISS used by the team, the Techshot Multi-Use Variable-gravity Platform (MVP), was not equipped to deliver and precisely control the needed carbon dioxide.
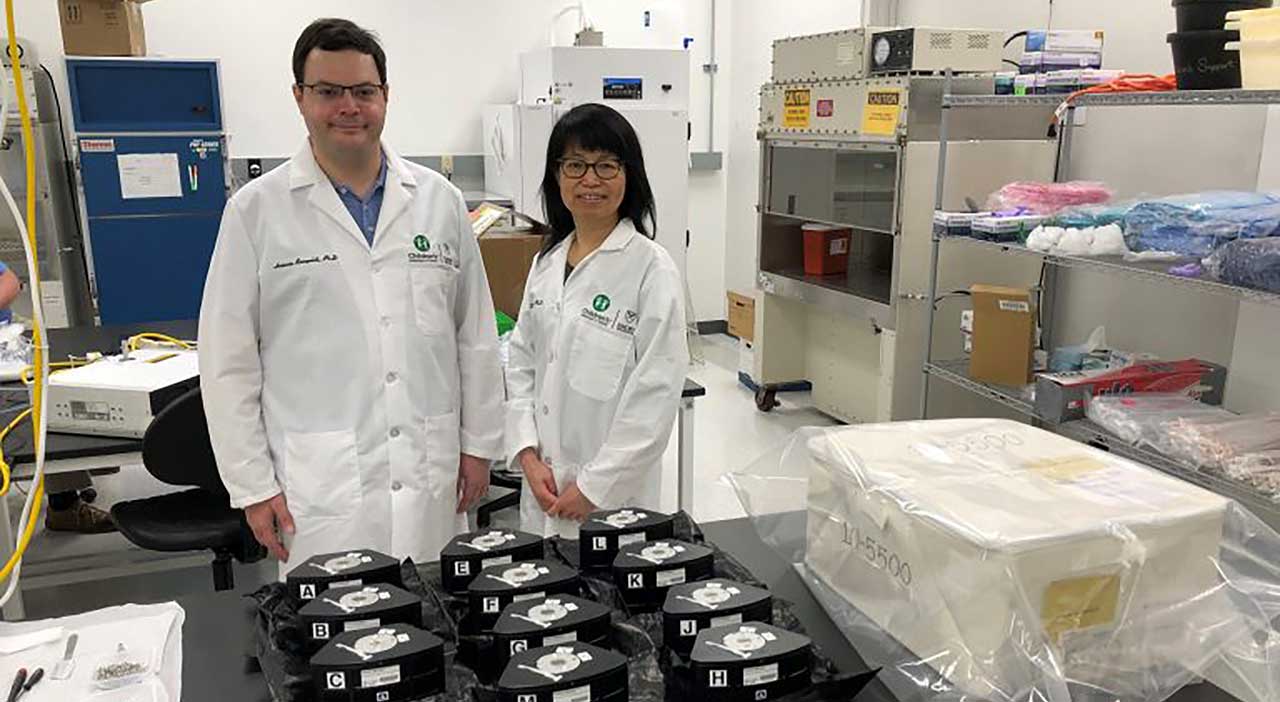
Chunhui Xu and Antonio Rampoldi, a postdoctoral fellow at Emory University, pack their cell culture hardware modules for the MVP Cell-03 investigation before launch.
Media Credit: Cardiomyocyte Stem Cell Laboratory, Emory University School of Medicine
Instead, Xu’s team created a novel growth medium by supplementing a commercially available carbon dioxide-independent substrate with a unique blend of additives, creating the perfect environment for cells to flourish without additional carbon dioxide.
“When we started the project, we didn’t expect to work on so many details to get spaceflight-ready,” Xu said. “We all recognized how important it was for this to be successful, because we knew we only had one shot.”
Proof of Concept
Xu’s experiment launched on SpaceX’s 20th Commercial Resupply Services mission(Abbreviation: CRS mission) A CRS mission is a cargo resupply mission contracted by NASA to deliver supplies and research to the International Space Station on commercial spacecraft as part of the CRS contract with three commercial companies. As part of CRS missions, experiments currently return to Earth on SpaceX Dragon spacecraft that splash down in the ocean. to the ISS, where the cells were thawed and cultured by crew members. When the cultures returned to Earth three weeks later, the team found their efforts had paid off.
“The cells not only survived in microgravity conditions but also turned into beating heart cells,” Xu said.
It was an important proof of concept: heart cells could be grown in space and returned to Earth in a viable, functioning state. Xu and her team published their results this past February in the journal Biomaterials, detailing their methods for cryopreservation and cultivation.
“We wanted to share this information because we thought it might be helpful to organ-on-a-chip studies or other types of research that deal with microtissues,” Xu said.
The study provided another set of important results: the cells exposed to microgravity outperformed controls, mirroring the results of the ground-based experiment. “The microgravity cultures did survive better and had higher proliferation capacity,” Xu said. “It was quite amazing.”
The extent of the effect is still under investigation, and Xu and her team are drafting a manuscript detailing the results. But the outcome was promising, she said.
Further study could help unveil the forces behind stem cell growth and survival, allowing researchers to speed up production and enhance the quality of heart cells for potential clinical applications.
“Eventually, the cells can be used for treating patients, uncovering new therapies, and testing potential drugs,” Xu said. “All of these applications rely on how efficiently we can control the cells.”
A One-of-a-Kind Place
Xu’s ISS National Lab-sponsored investigation was the subject of a recent virtual panel discussion, jointly hosted by Emory University and the Children’s Healthcare of Atlanta Heart Center. Joining Xu on the panel were Kevin Maher, another Emory researcher and director of the Heart Center’s cardiac intensive care unit, and 10-year-old heart patient Brody Parker.
Brody was celebrating the two-year anniversary of the heart transplant that saved his life after he was diagnosed with dilated cardiomyopathy, a dysfunction in the heart’s ability to pump blood. Maher said Xu’s study was an example of research that could someday be used to help patients like Brody.
“This type of work, to be able to really learn how to make cells grow better, has the potential to really increase the chance that that will happen someday,” Maher said during the panel. “It’s not a reality yet, but there’s a lot of work being done on seeing how we can use these types of stem cells to make the heart stronger and better in the future.”
The event also featured a special appearance by NASANational Aeronautics and Space Administration astronaut Jessica Meir, who participated in the delicate work of Xu’s experiment onboard the ISS.
Space is a unique environment that enables cutting-edge research that is not possible anywhere else, Meir said during the virtual event. “This one-of-a-kind place is what makes doing research on the International Space Station so special. We can test things that we can’t do anywhere on Earth,” she said. “Not only does this science help us understand more about living and working in space, but it also tells us more about life back here on Earth.”