From Viral Villain to Gene Therapy Hero: Assembling 3D Brain Organoids in Space to Advance Precision Neurological Treatments
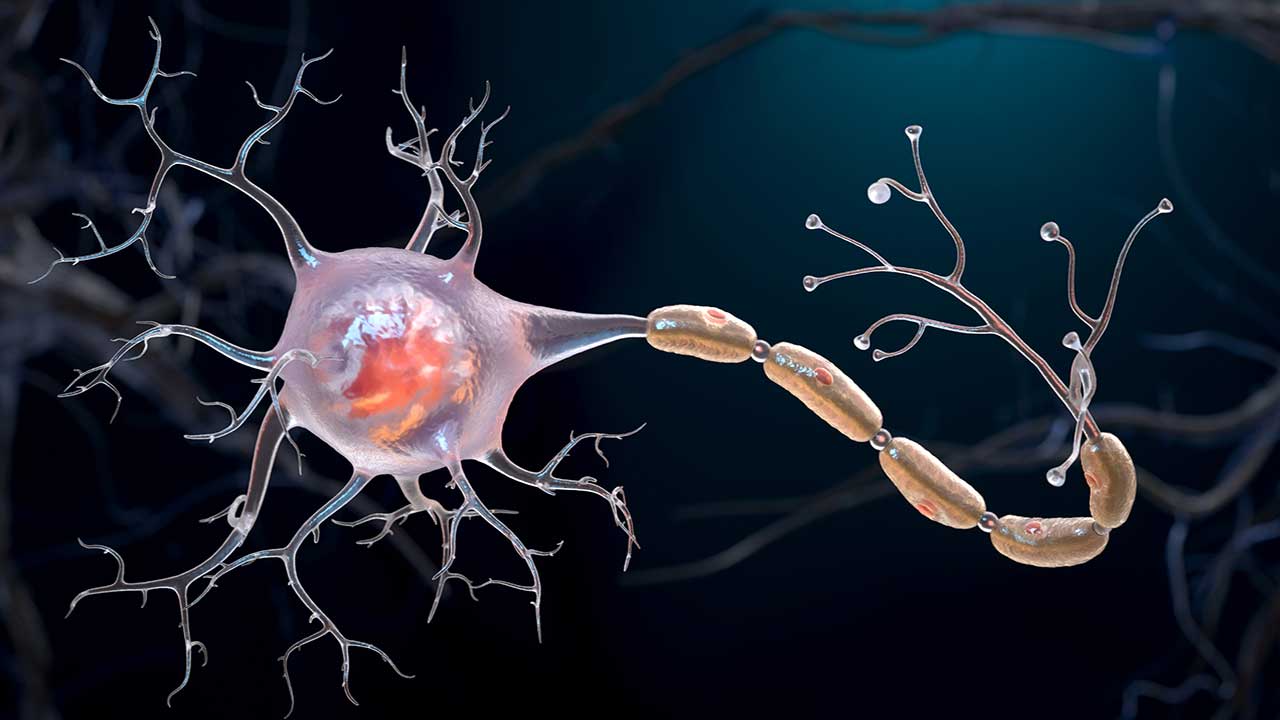
A neuron, or nerve cell, consists of a cell body, short extensions called dendrites, and a long arm called an axon that branches off into axon terminals. For messages to be sent from one neuron to another, an electrical signal travels down the axon, and the axon terminals pass an electrochemical signal to the dendrites of other neurons.
November 13, 2024 • By Amelia Williamson Smith, Managing Editor
In the classic dichotomy of hero versus villain, everything is black and white. Heroes are brave, noble, and the epitome of good. Villains are selfish, cruel, and downright evil. But, in reality, our world is much more complex, and everything is varying shades of gray—heroes have flaws, and villains can do good.
Most people think of viruses as villains. Viruses infect people, leading to bothersome symptoms like congestion, coughing, sneezing, and fever. In some cases, viruses can be more dangerous, such as HIV, the virus that attacks the immune system and causes AIDS. Other viruses, like the coronavirus that causes COVID-19, can spread quickly, causing severe illness and leading to a global pandemic. In these cases, viruses certainly act like villains.
However, sometimes viruses can be good—even heroic. Viruses have evolved to become very efficient at infecting cells in a host and inserting genetic material into the cells. In viruses that cause disease, this genetic material hijacks and damages cells. But scientists can reprogram some viruses to carry helpful, not harmful, genetic material. These modified viruses serve as messengers to carry beneficial gene therapies into cells to treat disease and injury.
One promising area is the use of gene therapies to treat currently incurable neurological conditions, such as Alzheimer’s, Parkinson’s, and spinal cord injuries. The problem is that gene therapies that work in rodent models often fail to translate into effective treatments for humans. So, there is a critical need for more accurate models of the human central nervous system (CNS)—which includes the brain and spinal cord—to test new therapies.
On Earth, researchers can generate human CNS cells from induced pluripotent stem cells (iPSCs), which typically are human blood or skin cells that have been reprogrammed. However, it is difficult to culture more than one neuron subtype together, and cell growth is limited to 2D monolayers because of gravity. This makes it challenging to create models that accurately mimic the diversity of CNS cells that function together in the human CNS.
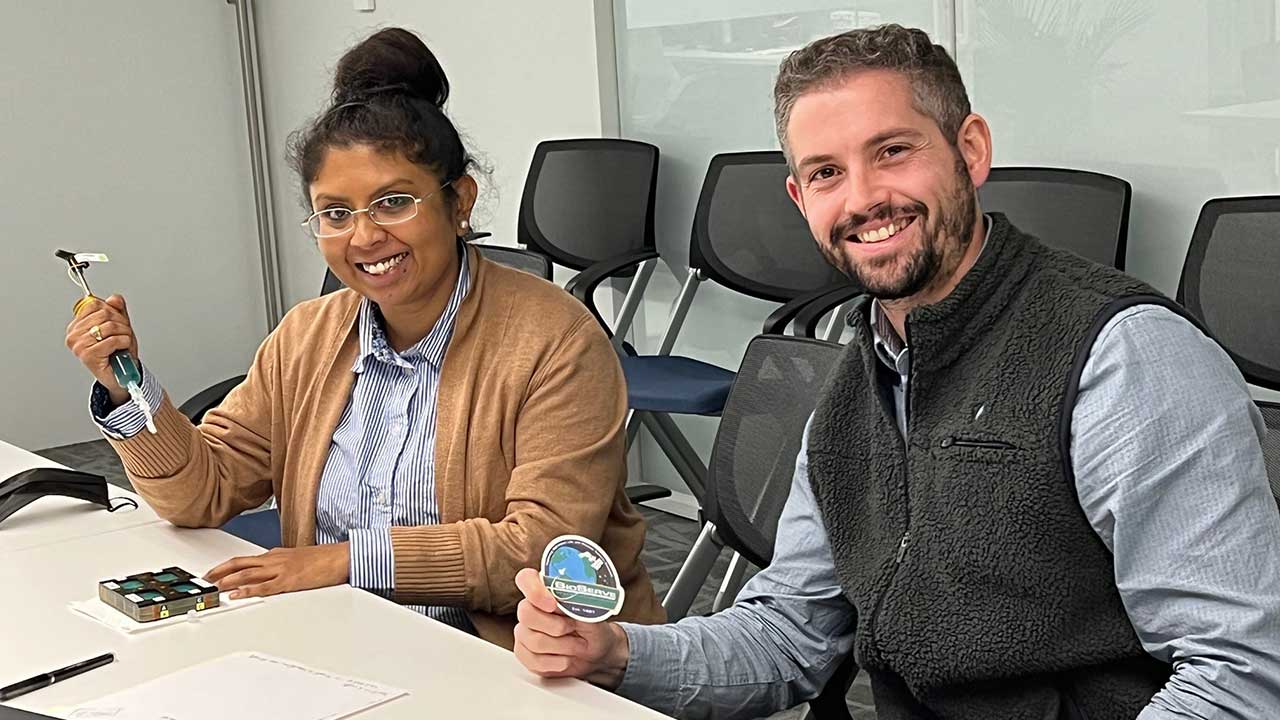
Axonis researchers Tia Dey and Shane Hegarty preparing for the team's ISSInternational Space Station National Lab-sponsored investigation.
Media Credit: Axonis Therapeutics and BioServe
Scientists at biotechnology startup Axonis Therapeutics wondered, “What if you take the human CNS cells to space, where gravity is removed?” Axonis had developed a viral vector designed to target neurons and deliver a novel gene therapy for neurological disorders. This therapy was designed to not only prevent neuron degeneration but also spur regeneration. The viral vector worked in mice, but would it work in a mature human brain model? Axonis needed to develop such a model to find out, and to do this, the company turned to the International Space Station (ISS) National Laboratory.
“Doing research in space is not something you’d ever think about normally, but the opportunity to leverage microgravityThe condition of perceived weightlessness created when an object is in free fall, for example when an object is in orbital motion. Microgravity alters many observable phenomena within the physical and life sciences, allowing scientists to study things in ways not possible on Earth. The International Space Station provides access to a persistent microgravity environment. conditions can unlock a lot of untouched potential by pushing the boundaries of science in a unique environment,” said Shane Hegarty, Axonis Therapeutics co-founder and chief scientific officer. “We wanted to see if we could overcome the limitations of ground-based iPSC-derived CNS models by co-culturing mature neurons and astrocytes in orbit and having them rapidly self-assemble into a 3D model of the human brain.”
Regeneration Rundown
The CNS is the most complex organ system in the human body. It allows us to think, feel, and take in the world around us through our senses. It keeps our lungs breathing and heart beating and allows us to move, communicate, learn, and remember. It’s how we solve complex problems and create beautiful things.
The CNS plays such a critical role in how we live and function that injury or disease involving the brain or spinal cord is often devastating and is currently irreversible.
“It is well known that peripheral nerves, which go from your spinal cord to the rest of your body, can regenerate,” said Axonis co-founder and scientific advisor Lisa McKerracher, who is also the founder and CEO of BioAxone BioSciences. “But for most of the 20th century, it was thought that any damage in the central nervous system would be permanent, and there was no chance to replace neurons or have them regenerate damaged axons.”
The Nitty Gritty of Neurons
The brain comprises two types of cells: neurons, which transfer messages, and glial cells such as astrocytes that support neurons and provide structure in the brain.
For a neuron to send a message to other neurons, an electrical signal travels down the neuron’s long axon. The axon branches off into axon terminals that pass an electrochemical signal to other neurons, and the process continues.
These messages travel down the CNS axons in the spinal cord to peripheral nerves, which are bundles of axons from different neurons grouped together. These nerves act like electrical wires that transmit messages throughout the body to control functions and sense our environment.
However, in the 1980s, McGill University neurologist Albert Aguayo, who would later become McKerracher’s postdoc mentor, was the first to demonstrate this is untrue. Aguayo showed that if you put a peripheral nerve graft into damaged CNS neurons, new axons will grow into the graft. He hypothesized that CNS neurons do not regenerate their axons because the environment around them isn’t conducive to regeneration.
After joining McGill University as a professor herself, McKerracher grew intrigued by a hypothesis from Swiss researcher Martin Schwab, who suggested it wasn’t just the cells’ environment that was a problem. Schwab proposed the existence of inhibitory proteins in the CNS whose purpose is to block cell regeneration. This hypothesis proved accurate, and McKerracher’s group was the first to biochemically isolate one such growth inhibitory protein. Since then, other inhibitory proteins have been discovered.
“The idea is that inhibitory proteins prevent sprouting of axon branches where you don’t want it to occur,” McKerracher said. “They keep the axons on their highway and don’t allow them to get distracted by going off on some other trajectory.”
However, this becomes problematic when a person has a spinal cord injury or a neurodegenerative disease that damages axons. The proteins prevent injured axons from regenerating, and damage is permanent.
Developing a Targeted Therapy
Axonis advisor Zhigang He at Harvard University discovered there are also intrinsic mechanisms within CNS neurons that play a key role in preventing axon regeneration. During brain development, CNS neurons grow their axons relatively long distances to form connections with other neurons in the CNS (for example, from the brain to spinal cord). However, in adults, expression of a gene called PTEN in CNS neurons acts as a master inhibitory protein within the neuron that suppresses the ability of axons to regrow after an injury.
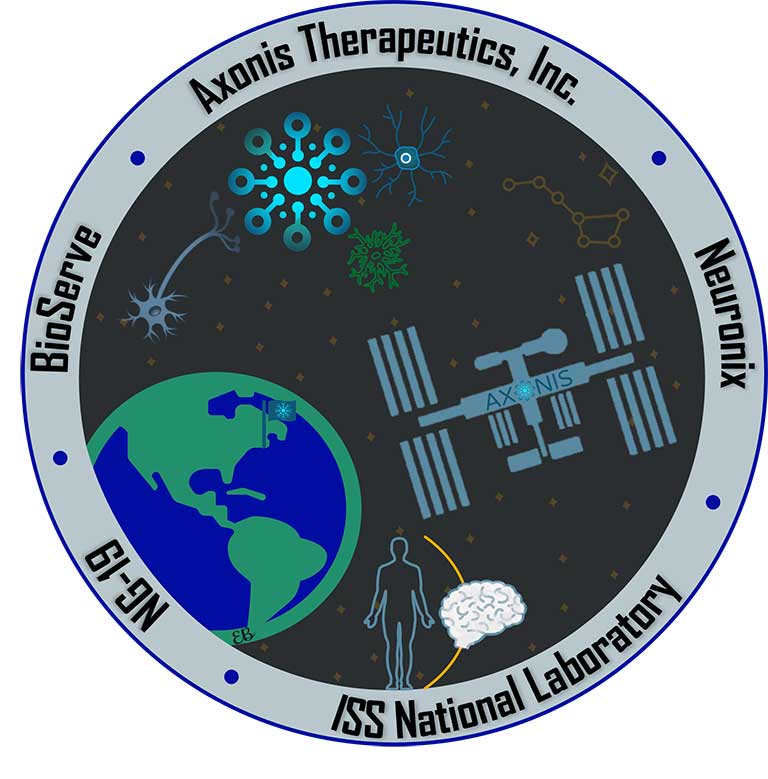
The mission patch for the team's ISS National Lab-sponsored investigation that was designed by Axonis team members Eilah Berlow, Tia Dey and Shane Hegarty.
Media Credit: Axonis Theraputics
Zhigang He was the first to show that a single gene manipulation, PTEN deletion, can directly reprogram CNS neurons back into a growth state that allows them to regrow their axons after injury. This groundbreaking discovery came from He’s Harvard Medical School and Boston Children’s Hospital lab and was spun off into Axonis.
To begin working toward a novel therapeutic to treat neurological conditions, Axonis developed a gene therapy that silences PTEN expression. But PTEN isn’t only expressed in neurons. The PTEN gene plays an important role in growth suppression in non-neuronal cells in the brain and in many cell types throughout the body. So, Axonis needed a way to target only CNS neurons with its neuroregeneration gene therapy.
The company used an adeno-associated virus (AAV), which does not cause human disease, to create a viral vector that infects cells and inserts the gene therapy in them. When the therapeutic gene is expressed in the cells, it turns off the PTEN gene.
Although the viral vector infects many different cell types in the body, Axonis found a way to limit the therapeutic gene’s expression to neurons by tailoring the vector’s gene promoter. A gene promoter is the DNA segment that comes just before a gene in a DNA strand and dictates the type of cells in which the gene can be expressed.
“What we did was design a vector that has a gene promoter only for CNS neurons,” Hegarty said. “Even though we knew the AAV would get into astrocytes and other cells, the therapeutic payload would not be expressed because that promoter is only active in neurons.”
When the team tested its neuron-specific AAV vector in rodent models, it successfully targeted only CNS neurons. The next step was testing it in a human brain model with both neurons and astrocytes.
Building a Better Model
When Axonis was just getting started in 2019, its CEO and co-founder, Joanna Stanicka, applied to the MassChallenge startup accelerator program. There, she learned about the Technology in Space PrizeA prize that provides grant funding for business startups participating in the MassChallenge startup accelerator program to conduct innovative research and technology development utilizing the ISS National Lab. The prize is funded by the Center for the Advancement of Science in Space, which manages the ISS National Lab, and Boeing., which provides startups with grant funding and the opportunity to conduct innovative research on the space station. The prize is funded by the Center for the Advancement of Science in Space™, which manages the ISS National Lab, and Boeing.
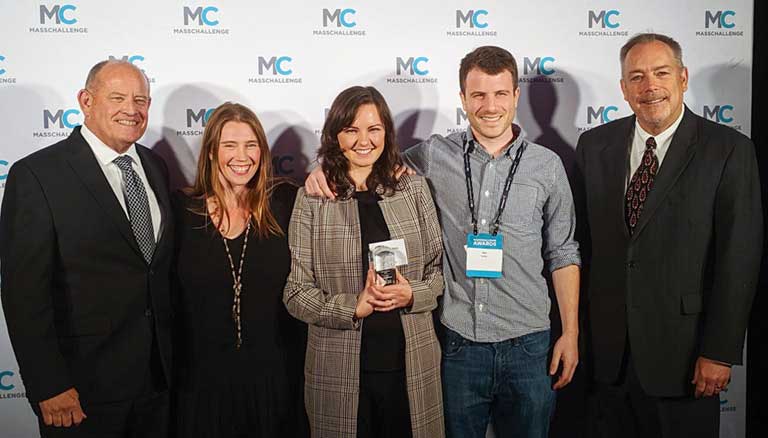
Through MassChallenge, Axonis was awarded a 2019 Technology in Space Prize from the ISS National Lab and Boeing. Axonis CEO, President, and Founder Joanna Stanicka (center) is pictured here receiving the award.
Media Credit: MassChallenge
“When the Technology in Space Prize was announced, our first thought was that we’re not a space company, so it’s not suitable for us,” Hegarty said. “But the opportunity encouraged our founding team to sit down and think, what could we do in space that would advance our company’s goals in terms of making new therapeutics for neurological disorders?”
Hegarty and his team came across a report from a research group that sent mature liver cells to space. The cells spontaneously assembled into organoids at a rapid pace in microgravity. This made them wonder if mature CNS cells would do the same.
Producing brain organoids on Earth is possible, but it is difficult and takes months. McKerracher explained that the process involves maturing iPSC-derived neurons and other CNS cells by using an artificial matrix substrate and growth factors. Even if you make brain organoids, the cells may not mature enough to accurately model adult CNS conditions and test drugs.
“You can take iPSCs and let them proliferate and differentiate into organoids, and these are powerful culture systems because you get 3D CNS models,” Hegarty said. “However, there’s a limitation on the maturity and on what types of cells you get—usually, if you only generate excitatory neurons, you won’t get any inhibitory neurons, so you don’t model that essential excitatory and inhibitory CNS neuron circuit. It is also a very lengthy culture process, which limits its scalability for drug screening.”
If Axonis could send fully mature neurons and astrocytes to space and have them rapidly self-assemble into a 3D organoid, it would provide a robust model for the company to demonstrate that its neuron-specific AAV gene therapy vector works in a human brain model. It would also significantly advance human brain modeling for future space-based research and therapeutic development.
“For studies in space, we wouldn’t need to send culture boxes with pre-grown organoids, which are impacted by launch and the flight to the ISS,” Hegarty said. “We could send various subtypes of cryo-preserved mature neurons and glia to the space station and then rapidly produce mature brain organoids in orbit, which would be extremely valuable.”
Adapting Science for Spaceflight
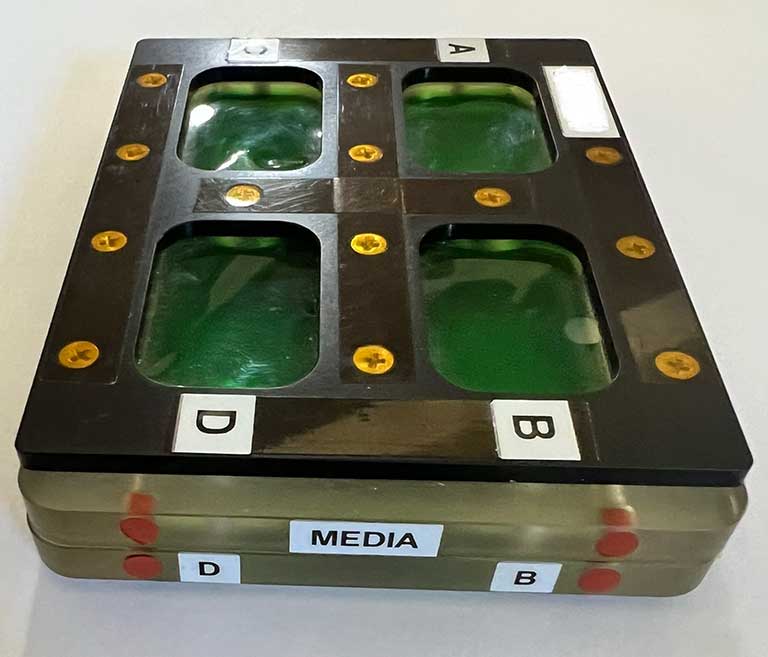
BioServe's four-well dual chamber BioCell hardware
Media Credit: BioServe Space Technologies
After submitting a proposal and being awarded the Technology in Space Prize, the Axonis team began working to develop their scientific concept into a spaceflight-ready project. To do this, the team partnered with ISS National Lab Commercial Service ProviderImplementation Partners that own and operate commercial facilities for the support of research on the ISS or are developing future facilities. BioServe Space Technologies.
“We work with research teams to understand their science, identify or develop hardware to support the science, and then help adapt their project to the hardware and the microgravity environment,” said BioServe research associate Sheila Nielsen.
The team used BioServe’s BioCell hardware, which has four wells per plate. The BioCell also has a large media chamber separated by a porous membrane that allows media to flow in but prevents cells from flowing out.
“The nice thing about our BioCell is that it provided a large environment for the cells to grow without attaching to the hardware, and it held enough media to feed the cells without having to do frequent disruptive media changes,” Nielsen said.
Axonis’ investigation launched to the ISS on Northrop Grumman’s 19th Commercial Resupply Services mission(Abbreviation: CRS mission) A CRS mission is a cargo resupply mission contracted by NASA to deliver supplies and research to the International Space Station on commercial spacecraft as part of the CRS contract with three commercial companies. As part of CRS missions, experiments currently return to Earth on SpaceX Dragon spacecraft that splash down in the ocean. in August 2023. The team sent the BioCell hardware prefilled with media, frozen vials of mature neurons and astrocytes, and frozen vials of the AAV vector. On station, the crew thawed the mature cells, injected them into the BioCell hardware, and then added in the thawed AAV vector.
Instead of loading the vector with Axonis’ gene therapy, the team loaded it with a fluorescent protein gene to visualize the cells. If the vector was successful and the fluorescent protein gene was only expressed in neurons, the neurons would glow green when the astronauts viewed the live human brain organoid cultures under a microscope.
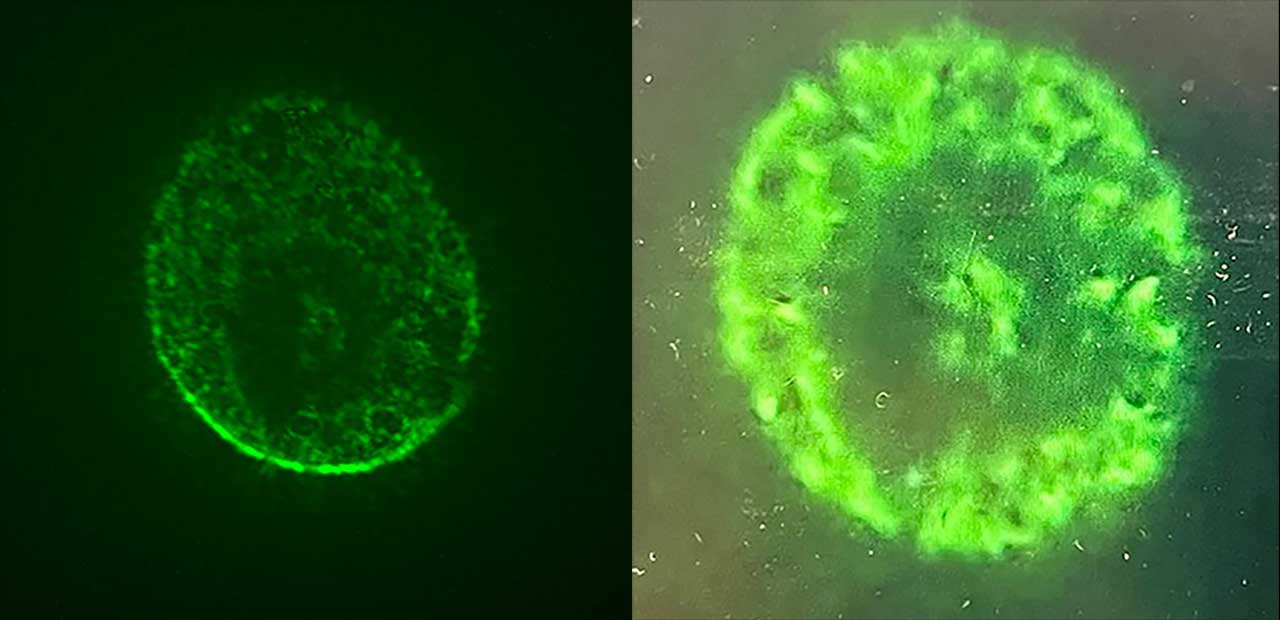
An image captured during in-orbit microscopy showing a human brain organoid model of iSPC-derived neurons and astrocytes expressing a neuron-specific AAV vector-delivered gene.
Media Credit: Axonis Theraputics
After 72 hours of letting the cells grow, the time had come to check on the cultures. BioServe communicated with the astronauts as they peered into the microscope, and anticipation ran high.
“The pivotal day was when the crew was doing the microscopy, and we saw big aggregates of green, glowing 3D tissue,” Nielsen said. “Shane and I were sending images of the organoids back and forth to each other, and we were pretty ecstatic because this is not something they had ever seen on the ground.”
Redefining Future Neurological Treatments
Over the course of 72 hours, the mature neurons and astrocytes self-assembled into 3D brain organoids. This was incredible, Hegarty said, because on Earth, mature neurons and astrocytes would die unless grown as a monolayer on a matrix substrate. Furthermore, the team could clearly see the bright green neurons, demonstrating that Axonis’ neuron-specific AAV gene therapy vector had worked.
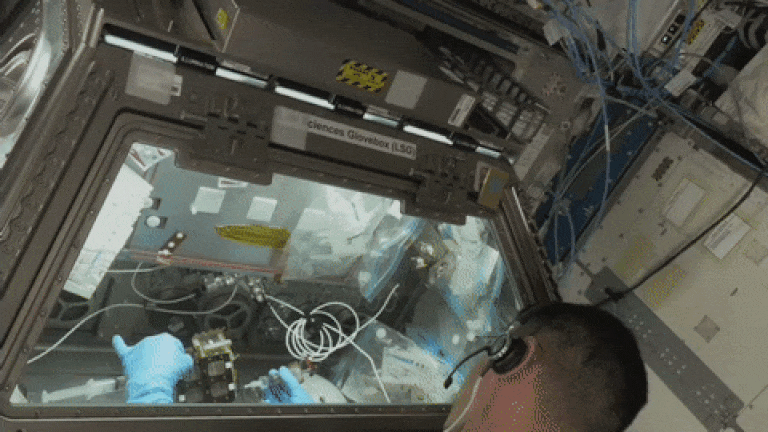
The ISS crew working on Axonis' investigation in the Life Sciences Glovebox on station.
Media Credit: NASANational Aeronautics and Space Administration
“It was very exciting—we were able to both validate the gene therapy targeting ability and show that we indeed rapidly formed 3D assembloid models of the human brain,” Hegarty said. “The major breakthrough is that you can self-assemble mature neurons and astrocytes rapidly into a model of the brain in microgravity conditions, which you can’t do on the ground.”
By successfully demonstrating the AAV gene therapy vector works in a human brain model, Axonis was able to significantly de-risk its therapeutic, helping to move it closer to clinical trials. Hegarty said the Technology in Space Prize also helped put Axonis on the map as a company. The grant made it easier for the startup to attract seed investors because it had its own non-dilutive capital and could hire its first research assistant.
Axonis’ results also have exciting implications for future space-based research. Hegarty says his team could take blood or skin cells from any patient and reprogram them to make the CNS cells relevant to that patient’s neurological disorder. The team could then combine these disease-relevant CNS cell types in microgravity cultures to rapidly develop a 3D model of the patient’s particular disorder.
“This would allow us to take a personalized medicine approach to developing novel, precision CNS therapeutics for individual patients and overcome current modeling limitations on Earth,” he said. “That’s the type of pioneering biotechnology research that can be done on the ISS now.”
Reflecting on his team’s success, Hegarty thinks all scientists should spend time thinking about space as a valuable research platform and how they could drive innovation by leveraging the transformational environment of the ISS.
“We never imagined it would be possible to advance our research in space, but the Technology in Space Prize gave us the opportunity to explore this possibility more deeply,” he said. “We now fully appreciate the amazing potential of performing research on the space station and have shown how you can leverage microgravity conditions to push your science beyond what’s currently possible on Earth.”