Microbes in Microgravity: Analyzing Gene Expression to Better Understand Bacterial Behavior in Space
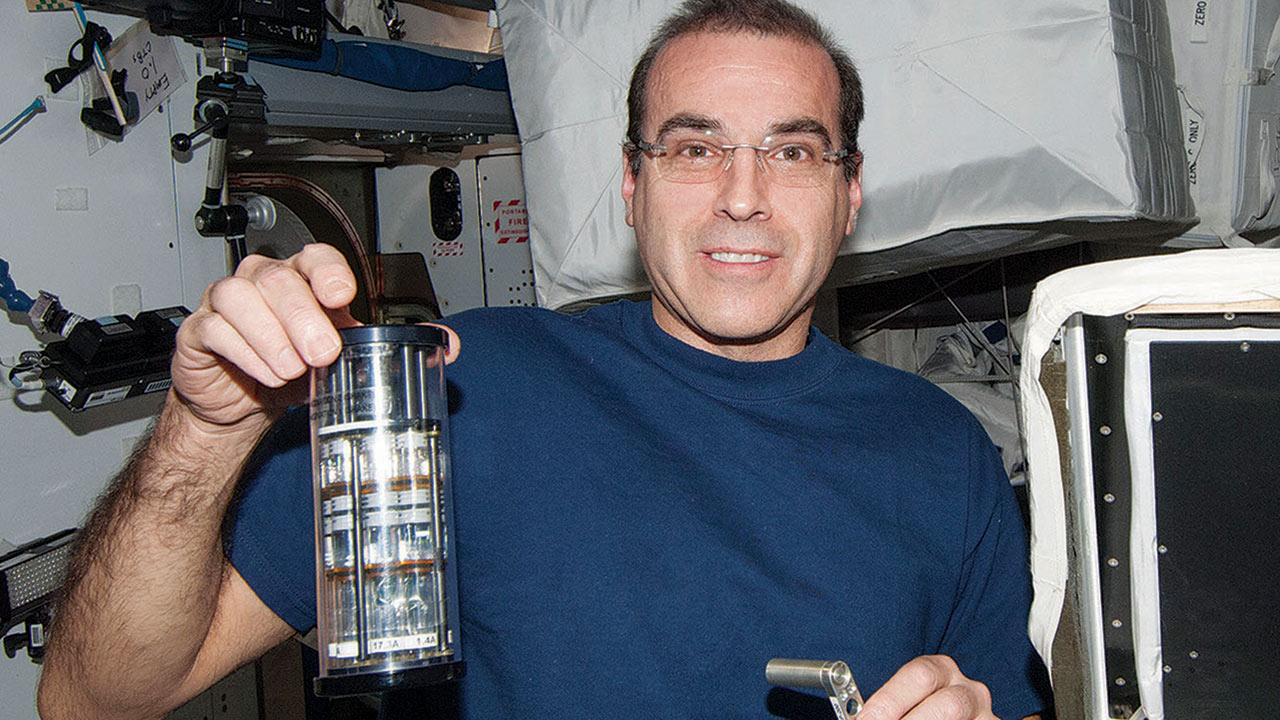
Media Credit: NASA
October 26, 2017 • By Amelia Williamson Smith, Staff Writer
By observing the health of astronauts that travel into space, scientists have learned that microgravityThe condition of perceived weightlessness created when an object is in free fall, for example when an object is in orbital motion. Microgravity alters many observable phenomena within the physical and life sciences, allowing scientists to study things in ways not possible on Earth. The International Space Station provides access to a persistent microgravity environment. has important effects on the human body, causing substantial changes to our bones and muscles. However, scientists have also found that microgravity has dramatic effects on far smaller living organisms, such as bacteria.
Bacteria behave differently in the microgravity environment of space than they do in a 1-g ground environment. For example, scientists have observed that in space, some strains of bacteria appear to exhibit enhanced growth and increased virulence (ability to cause disease). Additionally, higher doses of antibiotics are needed to kill some bacteria in space.
Scientists believe that these behavioral changes are not necessarily a direct effect of microgravity acting on the bacteria themselves— so what causes these changes in bacterial behavior?
Altering the Environment Around Bacteria
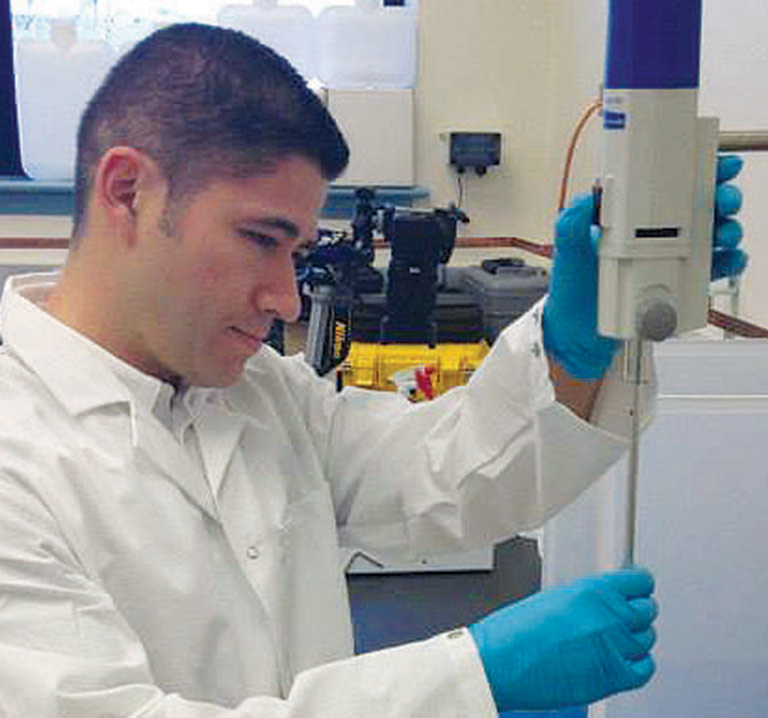
Dr. Luis Zea preparing the AES-1 experiment at Kennedy Space Center prior to the launch of Orbital ATK CRS-1.
Media Credit: BioServe Space Technologies
One model—the altered extracellular environment model—hypothesizes that the changes in bacterial behavior are actually an indirect effect of the microgravity environment, said Luis Zea, a researcher at BioServe Space Technologies at the University of Colorado, Boulder. The model postulates that microgravity alters the immediate environment around the bacterial cells, which then leads to changes in bacterial behavior.
Zea and his team conducted an experiment called Antibiotic Effectiveness in Space-1 (AES-1), in which they compared gene expression data from a nonpathogenic (not disease-causing) strain of Escherichia coli bacteria grown onboard the ISSInternational Space Station National Lab with cultures grown on the ground. AES-1 was launched to the ISS on Orbital ATK CRS-1 and returned to Earth on SpaceX CRS-3 and SpaceX CRS-4. Results from AES-1—published in the journal PLoS ONE in November 2016—strongly support the altered extracellular environment model.
“On Earth, there are different flows and forces, such as sedimentation, buoyancy, and convection, that don’t exist in space because they are gravity dependent,” Zea said. “The model states that it is the lack of these forces and flows that creates a different environment around the bacteria.”
“Through AES-1, we were able to corroborate, for the first time, this altered extracellular environment model that has been hypothesized for decades and could not be proven empirically or computationally,” Zea said. Although the AES-1 data only shows correlation and not yet causation, the results shed light on why bacteria behave the way they do in microgravity and open the door to further research on unicellular organisms.
Peeking Inside Bacterial Cells
On Earth, the movement of bacterial cells through their media is influenced by the physical properties of the medium, including gravity-driven forces like buoyancy and sedimentation, as well as other forces, such as the viscosity of the medium. As the cells move, they interact with fresh media and absorb molecules of nutrients. The cells also excrete waste products that may sediment down, float up, or trail behind the cells if they move, while simultaneously diffusing away.
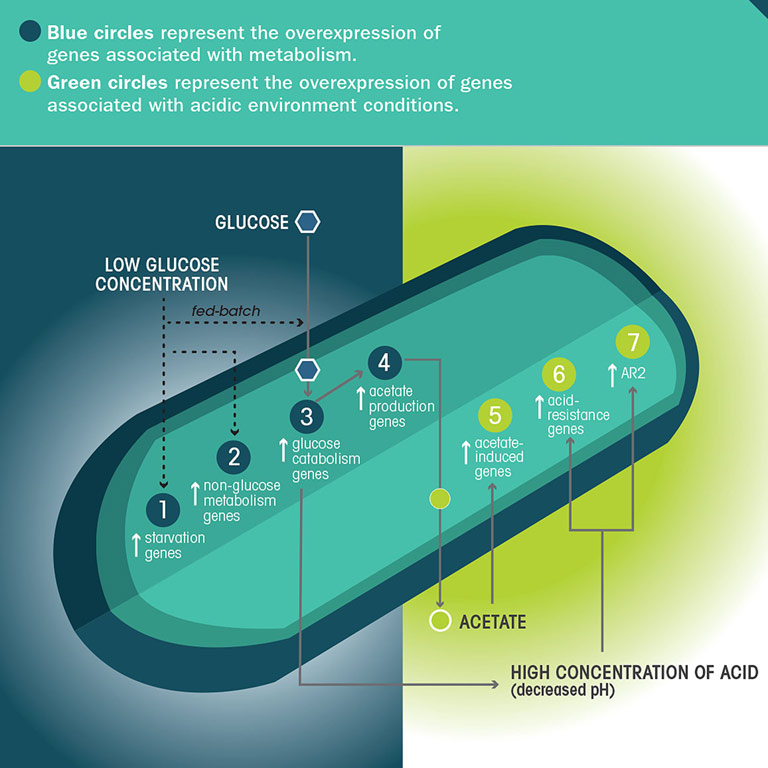
Media Credit: Zea L, Prasad N, Levy SE, Stodieck L, Jones A, Shrestha S, et al. (2016) A Molecular Genetic Basis Explaining Altered Bacterial Behavior in Space. PLoS ONE 11(11): e0164359.
However, in microgravity, these gravity-driven forces are absent, and the transportation of nutrients to cells and waste products away from cells are limited to diffusion-only transport. The altered extracellular environment model hypothesizes that the resulting reduction in the movement of molecules leads to less interaction of the cells with fresh media and thus reduced availability of nutrient molecules for absorption. Additionally, less movement causes waste products to accumulate around the cells, resulting in higher concentrations of potentially toxic compounds. The model postulates that these changes in the immediate environment around the cells are what lead, at least in part, to the changes in bacterial behavior observed in microgravity.
Researchers had tried to confirm the altered extracellular environment model using both physical measurement techniques and computational modeling; however, both methods fell short. Gene expression analysis gave researchers a new way to look at bacteria and test the model. If the model is correct, one would expect to see specific differences in gene expression in the bacteria grown in space versus ground controls, said AES-1 principal investigator David Klaus, professor at the University of Colorado, Boulder and faculty affiliate of BioServe Space Technologies.
“The gene expression data gives us a little peek inside the cell, which we have not had before,” Klaus said. “It is another layer that we’ve peeled back as we continue to try to figure out how bacteria respond to microgravity.”
Handling Test Tubes in Space
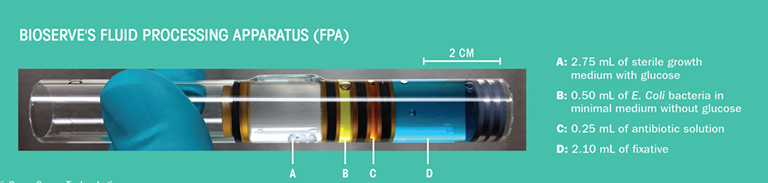
Media Credit: BioServe Space Technologies
For the AES-1 experiment, the research team prepared 128 bacterial culture samples to send to the ISS National Lab. The samples were each contained in a fluid processing apparatus (FPA), a test tube specially designed by BioServe Space Technologies for use in microgravity. Each FPA has four separate chambers—the first containing the growth medium with nutrients (glucose molecules), the second containing the E. coli bacteria, the third containing an antibiotic, and the fourth containing a fixative to preserve the sample for analysis back on the ground. Two different antibiotics were each tested under 16 conditions (different drug concentrations and fixatives), and all were tested in quadruplicate. Groups of eight FPAs were loaded into 16 BioServe Space Technologies group activation packs (GAP), which are cylinders that hold the FPAs and control the release of the solutions from the different compartments. Once the samples were onboard the ISS, a crew member used a crank to rotate the top of each GAP, introducing the bacteria in each FPA intothe growth medium to start the experiment. The bacteria were left to grow, and then the antibiotics of varying concentrations were introduced into the samples using the GAP.
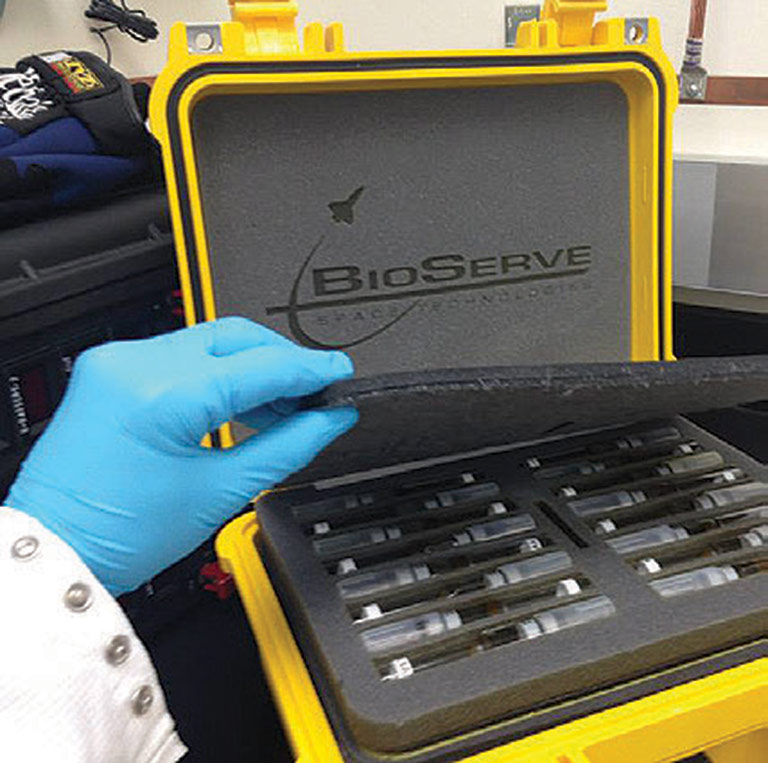
Media Credit: BioServe Space Technologies
“In space, you can’t just take test tubes and transfer the contents from one to another,” Klaus said. “The BioServe Space Technologies hardware allows us to first isolate the fluids and then sequentially mix them to start and stop the experiment within a single container without introducing the possibility of fluid leakage in the cabin.”
Once the AES-1 flight samples were returned to Earth, the research team performed gene expression analysis on the flight samples and ground controls at the HudsonAlpha Institute for Biotechnology in Huntsville, Alabama. This allowed the research team to examine—at a molecular level—the responses of the bacterial cells to their environment. The team compared the gene expression data from the microgravity-grown bacteria to the ground controls to determine if the flight results matched those predicted by the altered extracellular environment model.
Correlating Observations With the Model
If the model is correct, the microgravity-grown bacterial cells would have reduced interaction with fresh media and thus less nutrients available (even though the same amount of nutrients was provided for the flight samples and ground controls). Therefore, the researchers expected to see signs that the bacterial cells grown in microgravity were experiencing starvation conditions—and, indeed, they did.
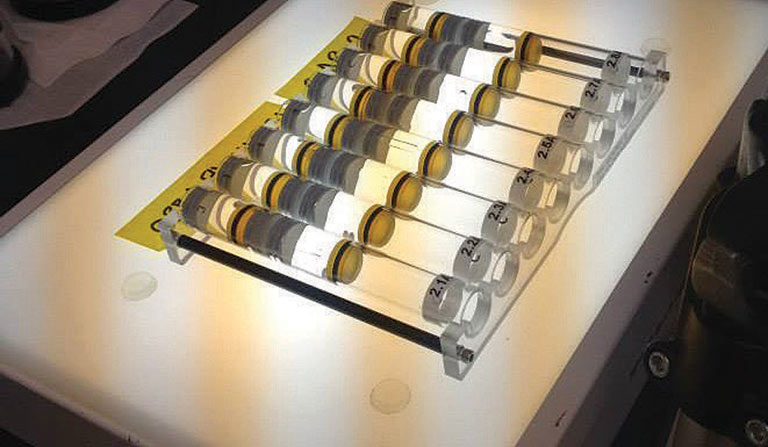
The researchers found an overexpression of genes in the microgravity-grown cells, relative to the ground controls, that indicate the cells grown in space experienced a lack of available nutrients. They also observed an overexpression of genes associated with the metabolism of other carbon sources (non-glucose nutrient molecules) that were not present in the growth medium. “It is believed bacteria do this to be able to change their metabolic pathways as soon as another carbon source becomes available,” Zea said. “This can be compared to the risk-prone foraging strategy that some animals use, in which they start engaging in high-risk behaviors looking for food.”
Analysis of the microgravity-grown bacteria also revealed an overexpression of genes associated with the metabolism of glucose, which may explain why some types of bacteria exhibit enhanced growth in space. Related to this, the team also found an increased expression of genes associated with acid production—acetic acid is a waste product of glucose metabolism.
It may seem counterintuitive that there was an increase in cell growth, given that the cells were under starvation conditions; however, this same phenomenon has been observed to happen on Earth under a very specific growth condition called fed-batch processing, Zea said. Scientists have found that higher bacterial cell counts occur when nutrients are absorbed incrementally (such as under starvation conditions), as opposed to when there is a steady absorption of nutrients (under normal batch conditions).
If the altered extracellular environment model is correct, the researchers also expected to see signs of higher concentrations of waste products, such as acetic acid, around the microgravity-grown cells due to the reduced mass transport of chemical compounds around the cells—and, again, they did.
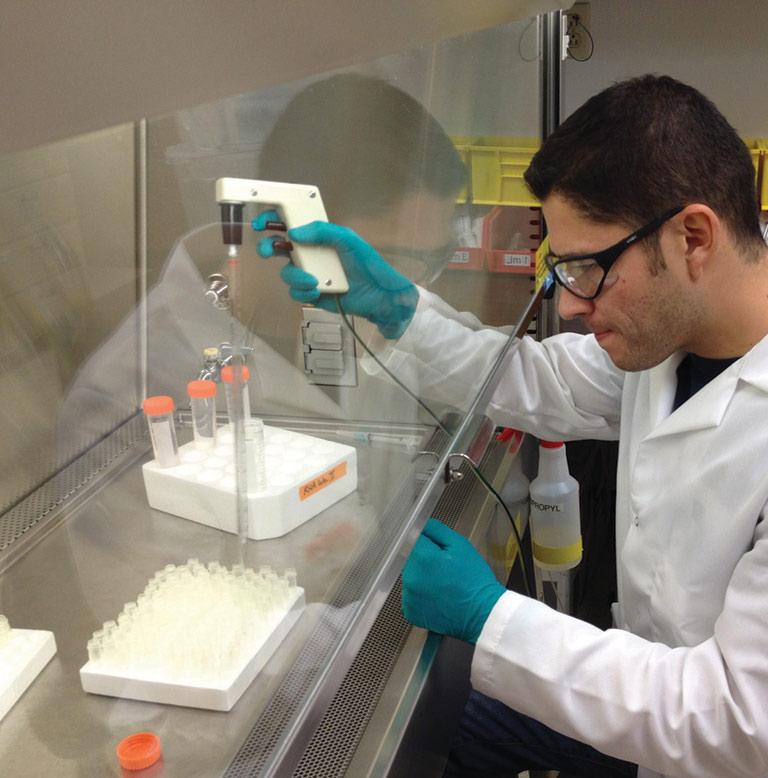
Media Credit: BioServe Space Technologies
In the microgravity-grown bacteria, the team found an overexpression of genes associated with acid resistance, suggesting increased acidity in the environment around the cells. However, the team did not observe differences in acidity of the bulk fluid around the cells in microgravity versus ground controls, suggesting the acidic environment in microgravity is limited to the immediate area around the cells. The buildup of acid may also explain the observed increase in virulence of some bacteria in space, as researchers have seen a correlation between an increase in acidity and an increase in virulence in certain bacterial strains, Zea said.
Together, these results provide strong support for the altered extracellular environment model. “I think the gene expression data was a real breakthrough,” Klaus said, “but it doesn’t prove cause and effect; it only shows correlation. Moving forward, we can begin to ask more definitive questions to get a much better understanding of what’s going on.”
Additional research is needed to confirm why higher concentrations of antibiotics are needed to kill bacteria in space. However, the altered extracellular environment model suggests that it may not be that bacteria have increased resistance to antibiotics in space, but instead, encounter less antibiotic due to the reduced concentration of antibiotic drug molecules around the cells.
“We’re trying to differentiate between bacterial drug resistance and bacterial susceptibility to drugs,” Zea said. “Is it that fewer drug molecules are reaching the cell due to the altered extracellular environment, thus physically reducing susceptibility, or is it that the cells are turning on resistance mechanisms more effectively? Or, it could be a mixture of the two, or something else altogether—that question is still open.”
Advancing Knowledge of Cells in Space
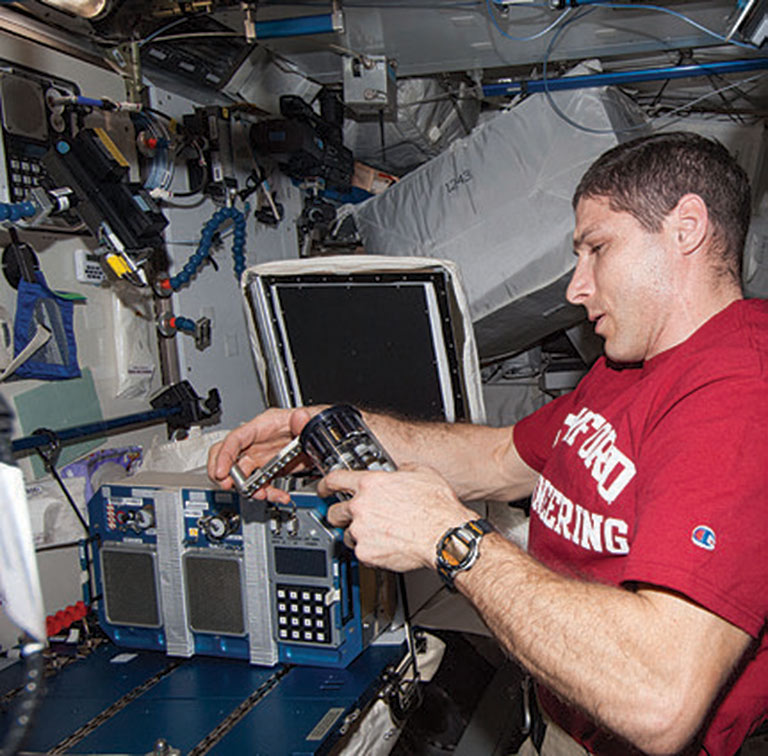
Media Credit: NASANational Aeronautics and Space Administration
The microgravity environment on the ISS National Lab allows researchers to probe the interactions between bacterial cells and their environment, and the resulting influences on bacterial behavior, in unique ways. “We’re trying to understand what’s going on at the interface of a cell and its environment,” Klaus said. “And in the absence of gravity, you can tease out some of these relationships in ways that are not really possible to recreate on Earth.”
Understanding changes in bacterial behavior in space is important not only because it helps better protect astronauts from infection during future long-duration spaceflight missions, but it also illuminates the mechanisms of bacterial behavior in our bodies on Earth. Additionally, an understanding of the mechanisms by which the altered extracellular environment in space triggers behavioral changes in microorganisms and our own cells informs research aimed at developing new vaccines, uncovering novel molecular targets against drug-resistant bacteria, and developing new antibiotics.
“There’s always an interplay between basic research and applied research, and they build on each other,” Klaus said. “So the more we understand the fundamental mechanisms, the more we can then use that knowledge to go after the real end goal—the development of application-oriented products.”