Spaceflight Studies for a Sustainable Future
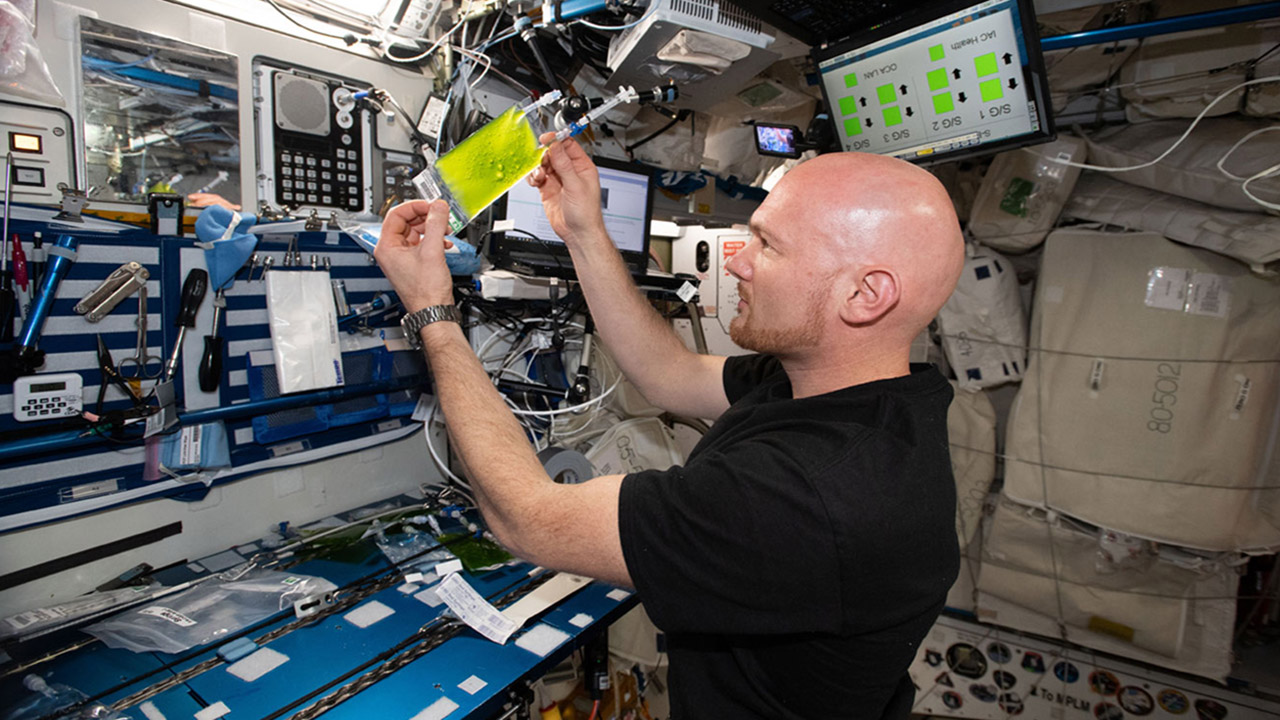
European Space Agency astronaut Alex Gerst preparing to transfer algae from a fully grown culture to new media.
Media Credit: NASA
July 11, 2019 • By Brian Greene
Humankind has made incredible technological advancements over the past two centuries. However, many of these advancements have come at a significant cost to the environment and risk to future generations. Globally, principal human impacts to the environment include accelerated changes to Earth’s atmosphere and climate, rising sea levels and increasing ocean temperatures, desertification, shortages of natural resources such as water and food, loss of biological diversity, and widespread pollution.
Now more than ever, it is important to address these issues, and scientists are leveraging the ISSInternational Space Station National Lab to gain new insights into global environmental challenges and develop new solutions to benefit Earth. Sustainability is a key focus area for research and development onboard the ISS National Lab, which currently has more than 30 sustainability projects in its portfolio.
ISS National Lab sustainability-related projects are diverse, including studies examining ecosystem production, sustainable land use, and water quality; remote sensing of our oceans and atmosphere for climate modeling; plant research to develop crops that require less water; and technology development designed to improve manufacturing methods and reduce harmful industrial emissions. Results from studies conducted onboard the ISS National Lab provide meaningful insights into materials and processes that drive sustainable development.
Three recent projects from the University of Florida; the University of California, Santa Barbara; and the Houston-based startup Cemsica showcase the diversity of sustainability research in the ISS National Lab portfolio.
Analyzing Algae Growth in Space
When people think of algae, they often imagine the green slimy substance that plagues aquarium tanks. However, algae are actually very useful. Algae can take carbon dioxide, water, and light and efficiently convert them into biomass, organic material that contains stored energy. Additionally, under certain types of stress, algae produce chemical compounds that have a variety of applications beneficial to humans, including medicine, fuel, and biodegradable plastics.
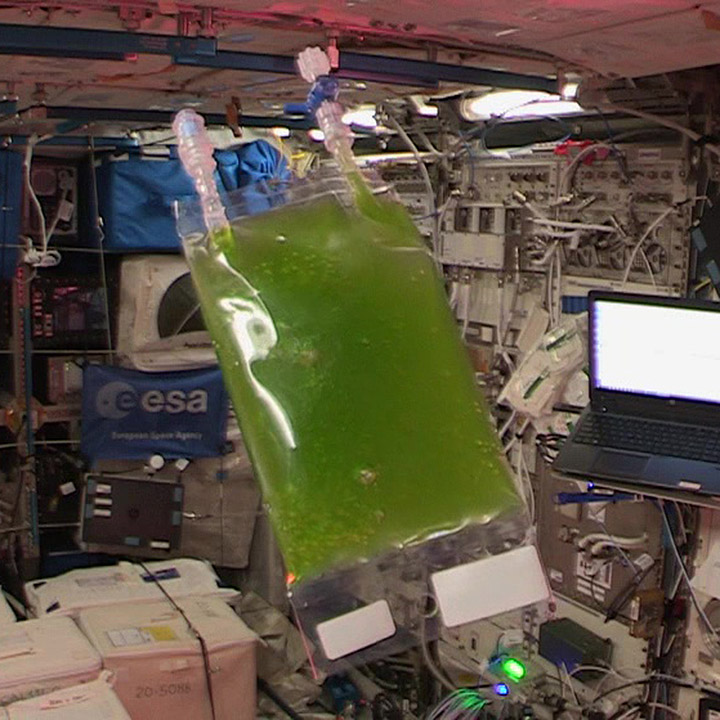
An algae bag floating in the ISS.
Media Credit: NASA
A research team from the University of Florida, led by Mark Settles, recently studied algae grown in microgravityThe condition of perceived weightlessness created when an object is in free fall, for example when an object is in orbital motion. Microgravity alters many observable phenomena within the physical and life sciences, allowing scientists to study things in ways not possible on Earth. The International Space Station provides access to a persistent microgravity environment. onboard the ISS National Lab. Understanding how algae behaves in microgravity—and whether this stressor enhances production of certain compounds—could advance efforts to genetically engineer algae with an improved ability to produce biomass and valuable compounds.
An important first objective of the experiment was to domesticate algae for growth systems that would be practical in space.
“On Earth, the systems used to grow algae efficiently rely heavily on gravity,” Settles said. “These methods are not applicable in a microgravity environment because you can’t rely on convection or the mixing of gas and liquid to provide the appropriate conditions for the algae to grow.”
Settles admits he was not confident that their initial experiment would even maintain a healthy population of algae.
Fortunately, when Settles and his team tested their algal growth system within the Veggie facility on the ISS, they found that it not only succeeded in maintaining a healthy population of algae but also resulted in production of more biomass than in ground controls. Settles posits that because algae do not settle into dense layers in space as they do on Earth, they were able to obtain greater access to the nutrients needed for growth.
These initial results are promising for two reasons. Firstly, the algae could possibly be cultivated as a space crop—both for nutritional use by humans and as a means to produce important compounds during long-term spaceflight. Secondly, results from the cultivation of algae with different genetic backgrounds in space could be used to advance efforts to grow a sustainable algae industry on Earth.
“Right now, to produce high-value compounds, we use fermentation of E. coli or yeast,” Settles said. “So, it’s taking resources that originally came from plants and converting them into things that microbes can use, as opposed to directly using light to produce want you want.”
Having a viable algae industry would reduce demands on land use and offer other environmental and cost benefits.
Settles and his team are still analyzing data from the spaceflight algae, looking for genetic variants that thrived in the space environment and evaluating whether the biomass contains beneficial compounds. Settles says follow-on experiments could include looking at other species of algae or genetically modifying the algae to try and improve them for human use. “If we could get to the point where we’re engineering algae to create custom compounds that are of high value, then these kinds of growth systems will be practical on Earth,” he said.
In this video from Seeker, Settles discusses his team’s ISS National Lab investigation to examine algae growth in microgravity (beginning at 1:07).
Video courtesy of Seeker
Studying Sediment Cohesiveness
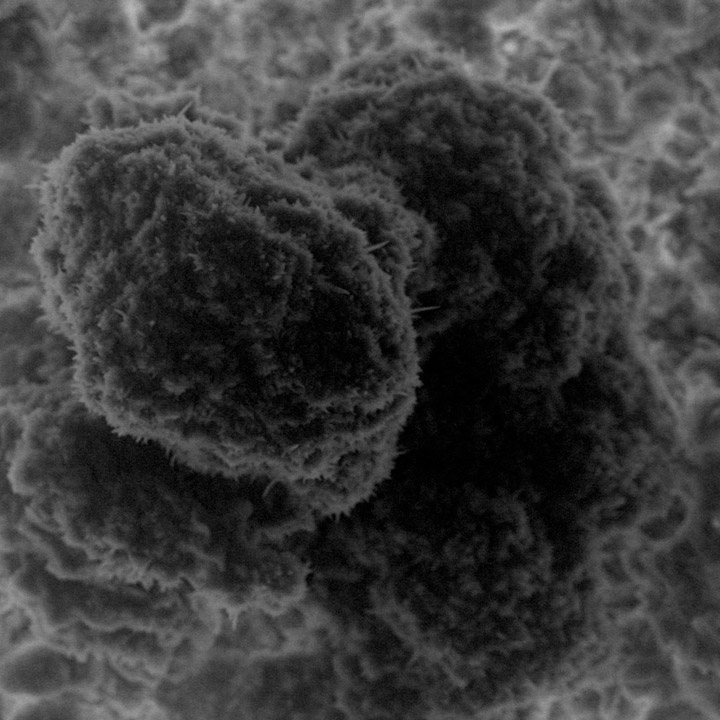
Clay aggregates viewed under an environment scanning electron microscope
Media Credit: UC Santa Barbara
In another recent ISS National Lab investigation, a team of researchers at the University of California, Santa Barbara, led by Paolo Luzzatto-Fegiz and Eckart Meiburg, used microgravity to examine the cohesive forces between sediment particles. The process by which sediment particles stick together influences their mobility in the environment and plays a central role in sediment transport in rivers, lakes, and oceans, which can have significant impacts on ecosystems and fisheries. Sediment settling is also an important factor in global modeling of the carbon cycle, as sediment near the surface of the ocean absorbs carbon from the atmosphere. However, it is difficult to study cohesive forces on Earth because of the complex interactions of sediment particles with gravity.
Ideally, to measure sediment cohesiveness, one would place sediment in a vial and stir it to break up any particle aggregates. Then, one could evaluate cohesiveness by examining how the particles re-clump together. But compared with the force of gravity, cohesive forces are very weak. Additionally, gravity-driven sedimentation causes particles to quickly clump and settle.
In the absence of gravity, particles aggregate much more slowly, allowing researchers to observe how they clump together and measure the cohesive forces involved.
“The idea is if you take this experiment to microgravity, you can run it for a very long time and get much greater detail about the aggregation process,” said Luzzatto-Fegiz. “Then you can figure out how cohesiveness depends on things like contaminants, salt content, and so on.”
A better understanding of how particles aggregate and the properties that can affect aggregation could lead to better predictive models of environmental systems.
Onboard the ISS National Lab, the team was able to observe sediment aggregation for a whole class of different sediment types and soil compositions, Luzzatto-Fegiz said. Moreover, the researchers had expected the aggregation to last one or two weeks before the large size of the particles would preclude useful data collection; however, the aggregation occurred over a much longer timeframe, allowing the team to collect detailed data for almost 100 days.
These data newly identified that the salinity of water plays a key role in the process of sediment aggregation. “That was really a fundamental contribution that came out of these space station experiments that was not realized before,” said Meiburg. Moreover, by comparing their spaceflight data with computer simulations, the research team produced numerical models of sediment transportation.
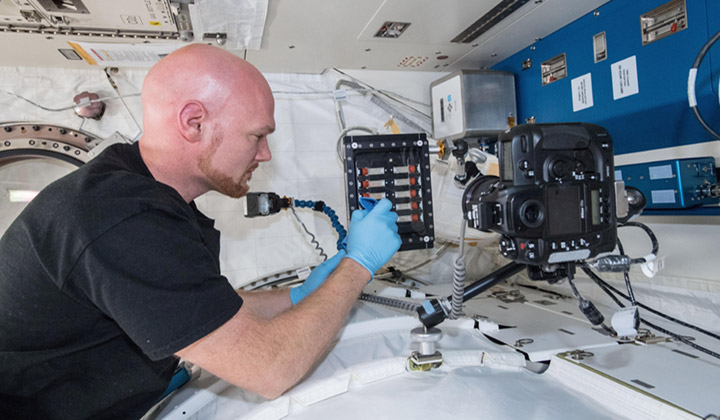
ESA astronaut Alexander Gerst onboard the ISS sets up the University of California, Santa Barbara experiment to examine the cohesive forces between sediment particles in microgravity.
Media Credit: NASA
The team published their results in the Journal of Fluid Mechanics in January 2019. “We presented a first-of-its-kind numerical simulation of cohesive sediment particles as they clump,” said Meiburg, “and we can demonstrate the mechanisms responsible for the fact that cohesive sediment settles out more rapidly than noncohesive sediment.”
These findings provide valuable information for several sustainability applications. “In the ocean, how fast sediment settles impacts how much light you get into a water column,” Luzzatto-Fegiz explained. “This in turn affects the ability of algae to bloom—and algae are the basis of the food chain in the ocean.” A reduction in sediment settling could lead to reduced algae growth, which can negatively impact ecosystems and fisheries.
Sediment settling also plays a significant role in the carbon cycle. Carbon dioxide in the atmosphere is absorbed by sediment near the surface of the ocean, and it is important to understand how quickly this sediment forms into larger particles that then settle down into the ocean, leaving the surface layer free to absorb more carbon dioxide. Understanding this process could aid the development of methods to help temper detrimental effects of rising levels of carbon dioxide in the atmosphere, which contributes to global climate change.
Carbon Cycle 101
Carbon compounds make up all living things, provide energy sources, and impact our climate. The global carbon cycle refers to the movement of carbon between the air, ground, water, and living matter. The oceans are the biggest reservoir of carbon on the Earth. Understanding the two-way exchange of carbon between the atmosphere and the ocean surface is particularly critical, as human-derived atmospheric carbon is on the rise.
Synthesizing Gas Separation Membranes in Microgravity
Despite many innovations in the energy industry, technology development has been lagging for the removal of gases, notably carbon dioxide, from waste air streams. The company Cemsica has been working on a potential breakthrough technology that addresses the critical need to reduce greenhouse gas emissions that result from industrial processes.
Cemsica is developing nanoporous membranes that can be tailored to remove specific contaminants, like carbon dioxide, from combustion exhaust gas streams, and the company recently conducted an investigation onboard the ISS National Lab aimed at improving synthesis of the membranes.
“The carbon dioxide footprint is one of the biggest problems in the energy industry,” said Negar Rajabi, founder and chief executive officer of Cemsica.
However, carbon dioxide is not always a waste product. It can also be a commodity sold as a feedstock for manufacturing chemicals and beverages—if the carbon dioxide is pure and can be recovered economically.
Commercial methods for capturing carbon dioxide have been around for several years but are complex and very expensive. As such, they have not been successfully implemented on a large scale. Cemsica believes its nanoparticle membranes are a better solution that will reduce operational costs, increase efficiency, and accelerate technological innovations in the industry that benefit the world.
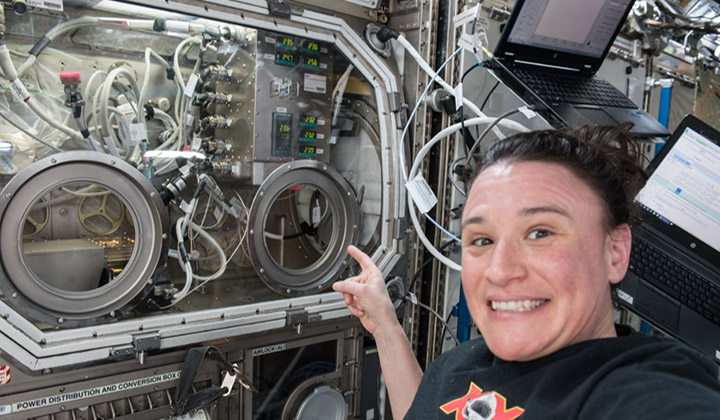
NASA astronaut Serena Auñón-Chancellor working on Cemsica’s investigation onboard the ISS.
Media Credit: NASA
A critical feature of Cemsica’s membranes are the nanopores—tiny holes that allow precise separation of carbon dioxide from other gas molecules. Because gravity significantly impacts how particles order themselves on the nanoscale, Rajabi hopes that synthesizing membranes onboard the ISS National Lab will allow Cemsica to overcome some remaining manufacturing challenges in the synthesis and assembly of the membranes—allowing them to produce even lower-cost membranes with improved properties.
Nanoscale Science
One nanometer—one billionth of a meter—is tens of thousands of times smaller than the width of a human hair. A nanomaterial is a material made up of many nanoparticles, which are 1 to 100 nanometers in size. Nanomaterials can be naturally occurring (e.g., volcanic ash) or engineered by humans.
Although nanomaterials are not new, Rajabi says there is still much to learn that can enhance nanomaterial properties. She and her team are still in the process of analyzing the data from their ISS National Lab investigation, but preliminary results have shown some improvements in the formation, shape, and size of the particles. Confirming these positive results would provide insights for improving manufacturing of the membranes on the ground and could also open the door for future in-orbit manufacturing in this sector, expanding industrial use of low Earth orbit(Abbreviation: LEO) The orbit around the Earth that extends up to an altitude of 2,000 km (1,200 miles) from Earth’s surface. The International Space Station’s orbit is in LEO, at an altitude of approximately 250 miles..
Looking After Our Planet’s Future
Achieving true sustainability has proven difficult and may require radical change, but novel approaches to sustainable living help preserve our Earth for future generations. Earth observations, life sciences investigations, and physical sciences studies onboard the ISS National Lab are each pushing the boundaries of innovation to help address many of our most pressing global environmental challenges.