Going Cool to Go Green: Studying Cool Flames in Space to Improve Engine Efficiency
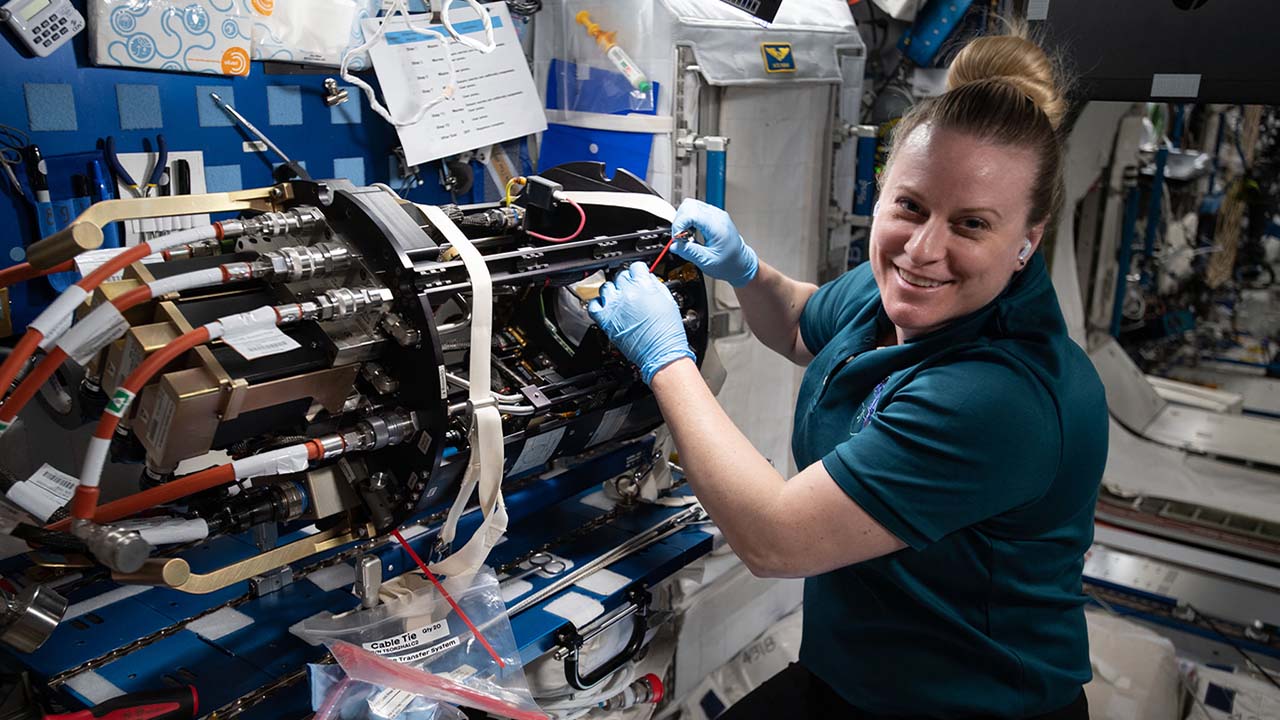
NASA astronaut Kate Rubins working on the cool flames investigation preparation.
Media Credit: NASA
June 29, 2023 • By Amelia Williamson Smith, Managing Editor
Transportation is crucial to our society, yet it is something most of us take for granted. There are more than 1.4 billion cars worldwide and approximately 100,000 flights that transport people and goods around the globe each day. People can get in their cars or hop on an airplane and travel wherever they want without really thinking about it. It’s hard to imagine life without the freedom of transportation.
It was a revolutionary invention in the late 1800s—the internal combustion engine—that forever changed how we travel and ushered us into the modern world of transport we enjoy today. However, it also brought with it the modern-day problem of pollution. With the climate change crisis we now face, there is an emerging need for new technology that makes internal combustion engines run more efficiently and reduces our carbon footprint.
To improve the efficiency of internal combustion engines and decrease pollutant emissions, scientists are turning to something that at first may seem like a contradiction of terms: cool flames. When you think of flames, the first thing that probably comes to mind is heat. Hot flames like those on a natural gas stove reach 3,100 degrees Fahrenheit. Cool flames, however, burn at much lower temperatures that typically do not exceed 1,000 degrees Fahrenheit.
For internal combustion engines, temperature plays a key role in both engine efficiency and the production of pollutants. If engines could be designed to incorporate aspects of cool flame chemistry, they would run cooler, meaning they could run cleaner and much more efficiently. But this requires an in-depth understanding of cool flame chemistry, which is difficult to study on Earth. Cool flame chemistry is slow, and gravity-driven buoyancy moves fast, extinguishing cool flames before they can fully establish.
To better study cool flames, scientists needed an environment where gravity, and thus buoyancy, is removed. This means they needed a laboratory not on Earth but in space. Leveraging the International Space Station (ISSInternational Space Station) National Laboratory, a team of researchers led by Peter Sunderland, a professor of fire protection engineering at the University of Maryland, set out to do something that couldn’t be done on the ground. In an investigation funded by the U.S. National Science Foundation, the team aimed to use microgravityThe condition of perceived weightlessness created when an object is in free fall, for example when an object is in orbital motion. Microgravity alters many observable phenomena within the physical and life sciences, allowing scientists to study things in ways not possible on Earth. The International Space Station provides access to a persistent microgravity environment. to produce a new type of cool flame never before seen that would provide valuable data to improve cool flame combustion models.
“On Earth, as soon as you have a flame, you get buoyancy, and the gases move so fast that the slow chemical processes can’t be observed,” Sunderland said. “But in space, you have a better environment where the molecules will hang around for a second or more inside the flame, and that’s when you can get this really slow, rare type of chemistry and record it and learn about it.”
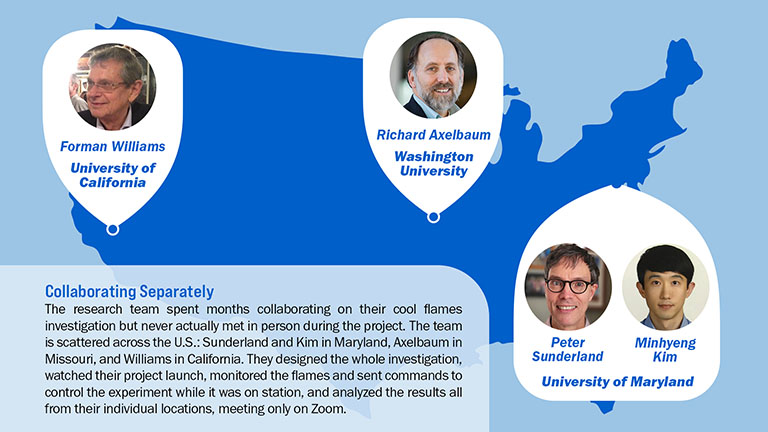
It All Comes Down to Temperature
Most cars with internal combustion engines burn gasoline at 35% efficiency. This means the average car is powered by only about one-third of the energy produced from engine combustion. What happens to the rest of the energy produced? The answer: it is lost to heat.
This loss from heat happens in two ways—hot exhaust that goes out of the car’s tailpipe, which not much can be done to avoid, and engine heat, which engine manufacturers are now focusing on. Current internal combustion engines run very hot, and a cooling system is required to lower the engine’s temperature for safe operation. High combustion temperatures also produce more nitrogen oxide (NOx) pollutants. If high combustion temperatures are the problem, cool flame chemistry could provide a solution, but not in the way you are probably thinking. Cooler combustion temperatures could be achieved not from cool flames themselves—the engine would still run on hot flames—but from incorporating cool flame chemistry that allows extra air to be added to the combustion.
In internal combustion engines, fuel is mixed with air at precise ratios and compressed, and a spark plug ignites the fuel-air mixture for combustion. If engine manufacturers could run the same amount of fuel through the engine but with twice the amount of air, it would run much cooler—so much cooler, in fact, that a cooling system may not be needed at all. Less energy would be lost to engine heat, and fewer NOx pollutants would be emitted.
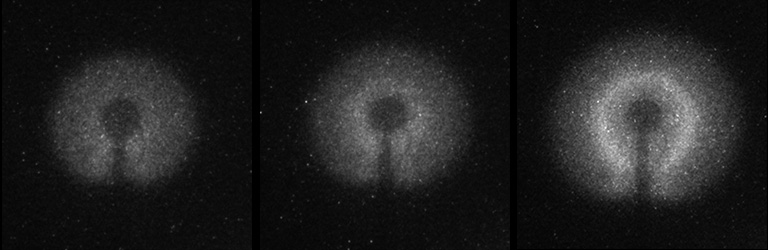
Intensified camera images of an n-butane cool flame onboard the space station. After the hot flame extinguished there was barely any luminosity (left). Then the glowing increased (center) until a steady cool flame was established (right).
Media Credit: University of Maryland/Peter Sunderland
However, for engines running with twice as much air, ignition with a spark plug would no longer work because the flames would extinguish or propagate through the fuel-air mixture too slowly, Sunderland explained. Instead, ignition would need to be through compression alone, which requires cool flame chemistry.
“In engines, ignition timing is essential,” Sunderland said. “With spark-ignited engines, the ignition timing can be controlled precisely if the flames are hot and strong. In future ultra-lean compression-ignition engines, the first reactions will involve cool flame chemistry, which will ignite the hot flames that propel the car.”
Currently, cool flame chemistry is poorly understood, and better computational models of cool flame chemistry are needed for engine manufacturers to be able to design compression-ignition engines.
“If we can master this cool flame chemistry, we could theoretically improve internal combustion engine efficiency from 35% to as high as 60%,” Sunderland said. “Many major car companies are now trying to understand cool flames to improve their technology.”
Discovering Cool Flames
The first detection of cool flames dates back to 1810 when British chemist Sir Humphry Davy accidentally discovered them while studying combustion to design safer lamps for coal miners. Two centuries later, in 2012, another accidental discovery—this time on the International Space Station—uncovered a different type of cool flame: cool diffusion flames.
Cool diffusion flames occur in systems where the fuel and oxidizer (oxygen or other oxidizing agent) are not premixed like the cool flames from 1810 were. Instead, the fuel and oxidizer diffuse toward each other, and the flame occurs at the place where they meet.
In the 2012 experiment, Forman Williams, professor of mechanical and aerospace engineering at the University of San Diego, was producing hot flames using liquid fuel droplets to study combustion in microgravity. When the hot flame went out, Williams and his team noticed the fuel droplet kept shrinking, which did not make sense. After much head-scratching, they realized that the hot flame had transitioned to a cool flame. Cool flames are so weak that they are barely visible and require a special intensified camera, which is why the researchers had not initially noticed them.
Building on the 2012 discovery, Sunderland had an idea. He joined forces with Williams and Richard Axelbaum, professor of energy, environmental, and chemical engineering at Washington University in St. Louis, who led a previous space station hot flames experiment Sunderland had worked on. Together, this power team of combustion researchers aimed to use the ISS National Lab to produce cool diffusion flames—not from liquid fuel like in the 2012 experiment—but from gaseous fuel.
Cool flames are prone to form with liquid fuels, which contain large molecules. But Sunderland and his team wanted to push cool flames to their limit. They wanted to find the smallest-molecule fuel that could be used to create a cool flame, which meant they had to go to gaseous fuels, Axelbaum said.
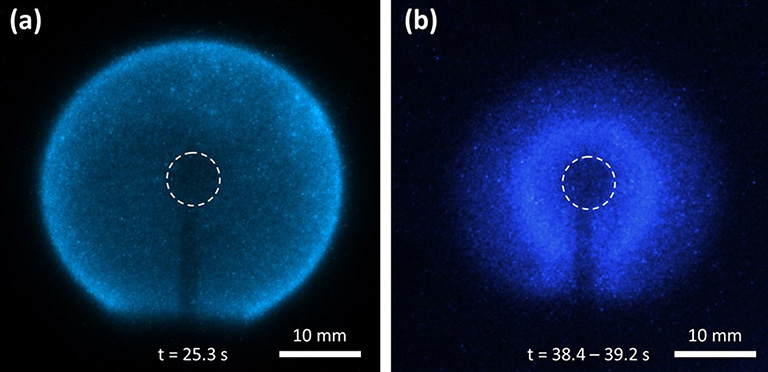
Intensified camera images from an n-butane hot flame on the space station (a) that transitioned to a cool flame (b). The original grayscale images have been converted to their expected blue colors. The times after ignition are shown. The dashed circles indicate the burner location. Image (b) is an average of images from the times shown and has an exposure 50 times as bright as that of (a).
Media Credit: University of Maryland/Peter Sunderland
The investigation used three gaseous fuels of decreasing molecular size: butane, propane, and ethane. These smaller-molecule fuels also make it easier to study cool flame chemistry, Sunderland explained.
“With big molecules, it’s much harder to understand the fundamental chemistry,” he said. “Smaller-molecule fuels like butane and propane provide a simpler system to work with. Gasoline is a really complex fuel, so getting a good understanding with the lighter hydrocarbons is the first step.”
What’s Gravity Got to Do With It?
Most people do not realize that gravity is critical to utilizing combustion on Earth. Think of a hot candle flame. When you light the candle, the flame establishes quickly and immediately travels upward to make the typical teardrop-shaped flame. This is because gravity-driven buoyancy causes the hot products of combustion to rise and this pulls in fresh air. It is this flow that keeps the flame going, and the process is very fast.
In contrast, cool flame chemistry is very slow, producing a weak flame that takes longer to establish. On Earth, buoyancy moves so quickly that it snuffs out cool flames almost immediately.
But in microgravity, flames burn much differently. Instead of taking on a teardrop shape, flames in space can be spherical. This happens because the only mechanism for the oxidizer to get to the flame is diffusion, which is weak and slow. In fact, it can be challenging to sustain hot flames in microgravity because there is no buoyancy to feed the flames quickly. But this is what makes space an ideal environment to study cool flames.
“If we have an environment where gases are moving quickly, and the chemistry is slow, it’s going to put out the flame,” Axelbaum said. “Cool flames involve really weak reactions, but in our microgravity experiments, we don’t disturb the flames at all, so that gave us hope that we could establish cool flames with our apparatus.”
Microgravity is beneficial for not only establishing cool flames but also sustaining them long enough for researchers to examine them.
“Without gravity, the flames don’t accelerate with buoyancy, so it can give you a longer time to study flame chemistry,” Williams said. “The International Space Station is a laboratory for learning more about the chemical kinetics of combustion that’s difficult to learn in experiments on the ground.”
The Search for a New Type of Cool Flame
On the space station, the experimental setup involved a sealed combustion chamber (about the size of a small office trashcan) filled with an oxygen and nitrogen mixture. Inside was a one-quarter-inch sphere made of sintered metal filings that allowed gas to flow through, and an igniter was used to start the combustion. The team tested the three fuels using different fuel and oxygen concentrations and different pressures. Their goal was to find the precise conditions needed to produce a cool diffusion flame from gaseous fuel.
While the experimental runs were happening on the space station, Sunderland and his graduate student, Minhyeng Kim, anxiously watched the flames from the ground through live video. “Watching the burning processes in the real-time videos was amazing,” Kim said. “It’s kind of beautiful—the colors, the shapes—and the flame glows bigger and bigger, and then suddenly it oscillates a little and is gone.”
Day after day, they watched the video and looked at the data, expecting to find hot flames transitioning to cool diffusion flames. In the first set of runs, they used ethane. In the next set, they used propane and, finally, butane. Each time, they watched the hot flame go out, waited several seconds, and then sent the command to stop the fuel flow. But day after day, run after run, the data showed no cool flames. By the time the butane experimental runs were nearly over, the team was discouraged.
“We thought we would easily get hundreds of cool flames, but we found that it was difficult,” Sunderland said. “I got home that night and went to bed feeling disappointed.”
Bothered by the fact that they had still not detected any cool flames, Kim did not go to bed. Instead, he poured through the day’s data. “I was reviewing the data and found that after the normal hot flame extinguished, there was still a little bit of signal coming from the heat sensor,” Kim said.
In the morning, Sunderland saw an email from Kim that was really exciting. “He had stayed up looking at the data and found the signature of a cool flame,” Sunderland said. “When we had stopped the butane flow, there had been a cool flame and we didn’t know it. It took us a few days to get the intensified camera images and the full data, but we were celebrating from then on—we had our first cool diffusion flame from gaseous fuel.”
Creating Higher-Accuracy Models
The ultimate goal of the investigation was to improve computational and numerical models of cool flame chemistry so engine manufacturers can design better internal combustion engines, Sunderland said. After successfully demonstrating cool diffusion flames using butane, the complex models had to be adjusted to account for smaller-molecule fuels.
Published data from the investigation allowed Williams and others to refine the chemistry in their models, adding important details that increased model accuracy. The data is helping to improve not only cool diffusion flame models but also premixed cool flame models.
“We had published the simplified chemistry, and now because of our results, we’re doing calculations with augmented simplified chemistry,” Williams said. “The new calculations point out that I had overlooked an important step that makes my published paper on premixed cool flame chemistry wrong, and I am correcting that.”
According to Axelbaum, the most exciting aspect is that the ISS National Lab allowed them to do something no one had ever done before and demonstrate that these types of flames can exist. “We gained a lot of knowledge we didn’t anticipate,” he said. “It’s wonderful to realize there are things you didn’t know, and you would never have known, if not for the unique opportunity to do these experiments in this novel environment.”
Notice of correction: The lead photo for this article has been replaced. The initial photo incorrectly showed an astronaut working on a previous cool flames investigation.